Coordinated progression through two subtranscriptomes underlies the tachyzoite cycle of Toxoplasma gondii - PubMed
- ️Fri Jan 01 2010
Coordinated progression through two subtranscriptomes underlies the tachyzoite cycle of Toxoplasma gondii
Michael S Behnke et al. PLoS One. 2010.
Abstract
Background: Apicomplexan parasites replicate by varied and unusual processes where the typically eukaryotic expansion of cellular components and chromosome cycle are coordinated with the biosynthesis of parasite-specific structures essential for transmission.
Methodology/principal findings: Here we describe the global cell cycle transcriptome of the tachyzoite stage of Toxoplasma gondii. In dividing tachyzoites, more than a third of the mRNAs exhibit significant cyclical profiles whose timing correlates with biosynthetic events that unfold during daughter parasite formation. These 2,833 mRNAs have a bimodal organization with peak expression occurring in one of two transcriptional waves that are bounded by the transition into S phase and cell cycle exit following cytokinesis. The G1-subtranscriptome is enriched for genes required for basal biosynthetic and metabolic functions, similar to most eukaryotes, while the S/M-subtranscriptome is characterized by the uniquely apicomplexan requirements of parasite maturation, development of specialized organelles, and egress of infectious daughter cells. Two dozen AP2 transcription factors form a series through the tachyzoite cycle with successive sharp peaks of protein expression in the same timeframes as their mRNA patterns, indicating that the mechanisms responsible for the timing of protein delivery might be mediated by AP2 domains with different promoter recognition specificities.
Conclusion/significance: Underlying each of the major events in apicomplexan cell cycles, and many more subordinate actions, are dynamic changes in parasite gene expression. The mechanisms responsible for cyclical gene expression timing are likely crucial to the efficiency of parasite replication and may provide new avenues for interfering with parasite growth.
Conflict of interest statement
Competing Interests: The authors have declared that no competing interests exist.
Figures
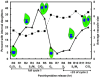
The average vacuole size (black boxes) and fraction of parasites containing internal daughters (black triangles) were determined in RHTK+ parasite cultures growth-inhibited by thymidine. At hour intervals through 12 h post-thymidine release, infected monolayers were fixed and stained with anti-IMC1. Vacuole size and the internal daughter fraction were determined in 100 vacuoles selected at random. The synchronous division of these populations is evident by the cyclical rise and fall of internal daughter forms that is accompanied by a stepwise increase in vacuole size that marks the end of one cycle and the start of the next. The cell cycle progression shown here was estimated from these experiments and is validated by previous studies using this synchrony model , .
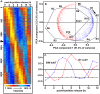
Duplicate RNA samples from each hour post-thymidine (0–12 h plus asynchronous samples) were used to synthesize cRNA probes for hybridization to the Toxoplasma Affymetrix GeneChip. Probe sets showing significantly increased variance through the cell cycle were identified by ANOVA analysis (10% false discovery) and further resolved by cubic B-spline modeling. (A) Heat map display of the 2,833 genes judged to be significantly cyclical was ordered by the times of the first principal maximum of RNA abundance in the first cell cycle, as estimated from the continuous cubic B-spline models of the time series for each gene. (B) Principal components analysis (PCA) provides objective evidence, following the logic described in the Results section, for the predominant cyclic expression series of the 2,833 genes (red and blue points) and the 13 time points (black), and for 2 predominant subtrancriptomes. Plots are superimposed of the 2-dimensional projections from PCA of the 2,833×2,833 between genes covariance matrix and the 14×14 between samples covariance matrix (the 13 time points R0 to R12 plus the asynchronous control labeled ‘Asyn’). The time axis circle runs clockwise, starting at the G1/S transition (R0) at the top, with the first S phase (R0-R2) corresponding to S phase in the second cycle (R8-R10) and the mitosis/cytokinesis of R4-R5 recurring at R11-R12. The cycle of synchronized sample points, especially in the partial second cycle, tends to converge slightly towards the ‘Asyn’ center as the time course proceeds, consistent with slight progressive loss of synchrony during the experiment. The red and blue gene points correspond respectively to the G1 and S/M subtranscriptomes, partitioned at 4.6 hours according to the criteria described in the Results section. (C) Singular value decomposition (SVD) analysis objectively reveals a cyclic process corresponding to the temporal G1 and S/M subtranscriptomes. Following the logic described in the Results and Methodssections, this analysis provides quantitative estimates of the RNA proportions, aggregated over genes, attributable to the non-cyclic steady-state expression level present throughout the cell cycle (component 1, black) and to the cyclically varying components of the two subtranscriptomes (components 2 and 3, red and blue). Smaller components of SVD, not shown, also identify small subsets of genes that have atypical profiles, but this analysis fails to find further major subclusters within the subtranscriptomes.
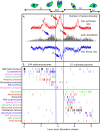
(A) Two major waves were apparent in the peak times of RNA abundance, corresponding to the S/M and G1 subtranscriptomes and separated by a transition phase of active RNA synthesis and decay. Histograms represent the numbers of genes (counted in 2-minute bins) showing maximum net increase in mRNA change (red), maximum RNA abundance (black), and maximum net rate of RNA decrease (blue, inverted scale) through the first complete parasite division cycle (0–8.7 h post-thymidine release). The maxima were computed from derivatives of the spline model curves (methods section). The peaks of RNA abundance (black histogram) showed the two major broad waves corresponding to the two subtranscriptomes. The genes in the black histogram S/M peak labeled a mostly showed maximum net decrease in mRNA levels in the later transition phase blue peak labeled a′. The genes in the black histogram G1 peak labeled b mostly derived from the prior transition phase red peak of maximum net increase in mRNA levels labeled b′. Thus, the transition phase is characterized by rapid increases and decreases in the amounts of many different transcripts, coincident with the internal budding and G1 initiation of daughter cells and the consumption of the mother cell. (B) The peak times of mRNAs in specific functional groups are partitioned serially into the two subtranscriptomes. The G1 Subtranscriptome comprised 1,146 probe sets, 54% of which can be annotated functionally by homology using current databases. The S/M-subtranscriptome contained 1,493 probe sets, of which 40% can be annotated functionally. Thus, the S/M subtranscriptome encodes a substantially greater proportion of ‘hypothetical proteins’ with no current functional homologs, which are either unique to Toxoplasma or conserved in other Apicomplexa. For 228 probe sets, the peak RNA abundance occurred within the 3.8–4.7 hour transition zone with proportions of functionally annotated versus hypothetical proteins resembling the S/M subtranscriptome.
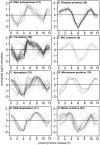
(A–D) Progression through the early G1 period is associated with maximum expression of transcripts encoding protein components of transcription and translational processes (5–6 h post-thymidine release) followed in mid-to-late G1 by a tightly clustering of genes involved in many aspects of DNA replication (7–8 h post-release). Apicoplastid genes (nuclear encoded) have a similar late-G1 profile consistent with the timing of plastid division in the next phase of the tachyzoite cell cycle (S phase). (E–H) Rhoptry and microneme proteins define distinct tight clusters with sequential timing during late S phase through mitosis. Rhoptry and IMC mRNA expression (35 total genes) peaked in late S phase/early mitosis (2–3 h post-thymidine release and then again at 10 h), which was followed by a rapid and dramatic decline of these transcripts in early G1. Transcripts encoding micronemes (10 genes) along with a number of motor proteins (kinesins and myosins; 23 genes) reach a maximum 1–2 h later as parasites undergo mitosis and complete budding (3–4 h and again at 11 h). The order of gene expression with plastid biogenesis coming before new rhoptry or microneme synthesis correlates with the timing of plastid and organelle replication during tachyzoite proliferation .
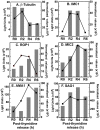
Protein accumulation roughly mirrors expression levels during the cell cycle. High protein levels are stably retained in R6 for microneme (MIC2, and AMA1) and rhoptry (ROP1) proteins despite down regulation of transcripts. Synchronized parasites were released and protein lysates generated at the times indicated. Levels of Toxoplasma proteins were detected by quantitative western blotting using specific antisera (see methods) and are shown as the average of three replicates (bar graphs). Normalized mRNA expression levels are shown from the microarray data (line plot).
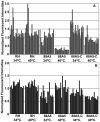
Single point expression levels for rhoptry (A) and microneme (B) mRNAs with cyclic RNA dynamics were examined in a temperature sensitive mutant that growth arrests in the early G1 period of the tachyzoite cell cycle (mutant 88A5; [38]). RHΔhxgprt- parent strain, ts mutant 88A5, and a genetically rescued 88A5 clone (88A5-C) were grown for 24 h at permissive (34°C) or non-permissive (40°C) temperatures. Duplicate RNAs were isolated and used to probe the Toxoplasma GeneChip. An expression heat map displaying the relative expression of all cell cycle genes in the mutant 88A5 and 88A5-complemented clone compared to the expression profiles of thymidine-synchronized tachyzoites is included in Figure S3. Normalized fluorescent intensities are shown for genes previously determined to have cyclic expression patterns (see Fig. 4E & G). Some variation (<2 fold) was detected between the parent and mutant strains in these experiments with rhoptry mRNAs having a larger range of abundance overall. However, the greatest difference in expression level observed was a consistent large decrease of the rhoptry mRNAs in mutant 88A5 parasites grown arrested at 40°C, which was rescued by genetic complementation.
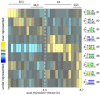
Over or underrepresented DNA motifs were identified in the 5′ regions of the 2,833 genes with cyclic mRNA profiles using the FIRE algorithm. For this plot, the intergenic regions interrogated were 0–2 kbp 5′-flanking to the coding regions, where the transcriptional start site predicted in ToxoDB (in some cases this is the ATG codon) was used to set 0 bp position. Intergenic regions were ordered by the peak time of mRNA abundance during the first complete tachyzoite cell cycle (identical order in Figs. 2A and Figs.3). Informative DNA motifs (rows) that are over or underrepresented in cohorts of similarly timed genes are shown (∼100 genes per vertical block; one complete cycle). No significant DNA motifs were detected if the expression order was randomized nor were DNA motifs found in the intergenic windows 2–4, 4–6, or 6–8 kbp 5′-flanking this gene set.
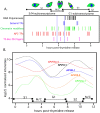
Cell cycle expression profiles were assessed for 68 predicted AP2 domain genes and 9 genes containing C2H2 Zn-finger domains (TGME49_103050, _121330, _101340, _086710, _006650, _002470, _059650, _023570, _111450) found in the Toxoplasma genome. (A) Maximum expression of RNA polymerases was tightly clustered in the G1-subtranscriptome along with a few general transcription factors. By contrast, the times of peak expression for 24 AP2 (see full list Table S1) and four activator Zn-finger domain genes were distributed across the tachyzoite division cycle (Table S2). In comparison, mRNAs encoding RNA polymerases showed peak expression clustered in G1. Relatively few general transcription factors and less than a third of known chromatin remodelers also have cyclical patterns (see also Table S2). (B) Spline model curves for selected cell cycle AP2 mRNAs demonstrate relative abundance and time shifts indicating that the expression of these factors follows a serial order with peaks at different cell cycle stages.
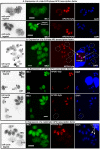
The endogenous loci of five cyclically expressed AP2 genes were epitope tagged with YFP in RHΔku80 . All tagged AP2 proteins were localized to the parasite nucleus. (A) AP2VIIa-4yfp was expressed beginning in G1 with peak expression in S phase (B) followed in time sequence by AP2VI-1yfp, which has an exclusive S phase expression profile. Merge inset indicates nuclear co-localization. (C and D) Three AP2 proteins had maximum expression in mitosis; AP2XI-1yfp and AP2XII-9yfp, while AP2VIIa-1yfp (D) was expressed in parasites that had divided their nucleus or were newly emergent from the mother. By mid-G1, AP2VIIa-1yfp was undetectable (not shown). Note; there is little expression of AP2VIIa-1yfp detected in parasites that were undergoing nuclear division (u-shaped nucleus) suggesting that the induction of this factor may be coupled to the completion of telophase. Co-stains: anti-IMC1 (green); anti-YFP (red), genomic DNA (DAPI, blue). Cell cycle legend is decolorized anti-IMC1 staining (inverted image) with labeling of internal daughters and cell cycle phases: G1 and S phase, C = cytokinesis, M = mitosis. Dashed circles denote the lack of AP2yfp expression, while the relative magnification is referenced by a 5 µm white bar.
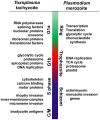
Similar articles
-
The Toxoplasma Centrocone Houses Cell Cycle Regulatory Factors.
Naumov A, Kratzer S, Ting LM, Kim K, Suvorova ES, White MW. Naumov A, et al. mBio. 2017 Aug 22;8(4):e00579-17. doi: 10.1128/mBio.00579-17. mBio. 2017. PMID: 28830940 Free PMC article.
-
Novel CRK-Cyclin Complex Controls Spindle Assembly Checkpoint in Toxoplasma Endodyogeny.
Hawkins LM, Naumov AV, Batra M, Wang C, Chaput D, Suvorova ES. Hawkins LM, et al. mBio. 2021 Feb 22;13(1):e0356121. doi: 10.1128/mbio.03561-21. Epub 2022 Feb 8. mBio. 2021. PMID: 35130726 Free PMC article.
-
Loss of the Conserved Alveolate Kinase MAPK2 Decouples Toxoplasma Cell Growth from Cell Division.
Hu X, O'Shaughnessy WJ, Beraki TG, Reese ML. Hu X, et al. mBio. 2020 Nov 10;11(6):e02517-20. doi: 10.1128/mBio.02517-20. mBio. 2020. PMID: 33173004 Free PMC article.
-
Host sensing and signal transduction during Toxoplasma stage conversion.
Augusto L, Wek RC, Sullivan WJ Jr. Augusto L, et al. Mol Microbiol. 2021 May;115(5):839-848. doi: 10.1111/mmi.14634. Epub 2020 Nov 21. Mol Microbiol. 2021. PMID: 33118234 Free PMC article. Review.
-
Lytic Cycle of Toxoplasma gondii: 15 Years Later.
Blader IJ, Coleman BI, Chen CT, Gubbels MJ. Blader IJ, et al. Annu Rev Microbiol. 2015;69:463-85. doi: 10.1146/annurev-micro-091014-104100. Epub 2015 Aug 28. Annu Rev Microbiol. 2015. PMID: 26332089 Free PMC article. Review.
Cited by
-
Hehl AB, Basso WU, Lippuner C, Ramakrishnan C, Okoniewski M, Walker RA, Grigg ME, Smith NC, Deplazes P. Hehl AB, et al. BMC Genomics. 2015 Feb 13;16(1):66. doi: 10.1186/s12864-015-1225-x. BMC Genomics. 2015. PMID: 25757795 Free PMC article.
-
ApiAP2 transcription factor restricts development of the Toxoplasma tissue cyst.
Radke JB, Lucas O, De Silva EK, Ma Y, Sullivan WJ Jr, Weiss LM, Llinas M, White MW. Radke JB, et al. Proc Natl Acad Sci U S A. 2013 Apr 23;110(17):6871-6. doi: 10.1073/pnas.1300059110. Epub 2013 Apr 9. Proc Natl Acad Sci U S A. 2013. PMID: 23572590 Free PMC article.
-
Pasquarelli RR, Sha J, Wohlschlegel JA, Bradley PJ. Pasquarelli RR, et al. PLoS Pathog. 2024 Jul 18;20(7):e1012411. doi: 10.1371/journal.ppat.1012411. eCollection 2024 Jul. PLoS Pathog. 2024. PMID: 39024411 Free PMC article.
-
Heredero-Bermejo I, Varberg JM, Charvat R, Jacobs K, Garbuz T, Sullivan WJ Jr, Arrizabalaga G. Heredero-Bermejo I, et al. Mol Microbiol. 2019 Jan;111(1):46-64. doi: 10.1111/mmi.14138. Epub 2018 Nov 28. Mol Microbiol. 2019. PMID: 30362624 Free PMC article.
-
Li ZY, Lu J, Zhang NZ, Elsheikha HM, Hou JL, Guo HT, Zhu XQ. Li ZY, et al. Parasite. 2018;25:37. doi: 10.1051/parasite/2018040. Epub 2018 Jul 23. Parasite. 2018. PMID: 30040611 Free PMC article.
References
-
- Chotivanich K, Udomsangpetch R, Simpson JA, Newton P, Pukrittayakamee S, et al. Parasite multiplication potential and the severity of Falciparum malaria. J Infect Dis. 2000;181:1206–1209. - PubMed
-
- McDonald V, Shirley MW. The endogenous development of virulent strains and attenuated precocious lines of Eimeria tenella and E. necatrix. J Parasitol. 1987;73:993–997. - PubMed
-
- McDonald V, Shirley MW, Bellatti MA. Eimeria maxima: characteristics of attenuated lines obtained by selection for precocious development in the chicken. Exp Parasitol. 1986;61:192–200. - PubMed
Publication types
MeSH terms
Substances
Grants and funding
LinkOut - more resources
Full Text Sources
Other Literature Sources
Molecular Biology Databases
Research Materials