No time to lose--high throughput screening to assess nanomaterial safety - PubMed
Review
doi: 10.1039/c0nr00618a. Epub 2011 Feb 7.
S George, M Li, S Pokhrel, Z Ji, B France, T Xia, E Suarez, R Rallo, L Mädler, Y Cohen, E M V Hoek, A Nel
Affiliations
- PMID: 21301704
- PMCID: PMC3980675
- DOI: 10.1039/c0nr00618a
Review
No time to lose--high throughput screening to assess nanomaterial safety
R Damoiseaux et al. Nanoscale. 2011 Apr.
Abstract
Nanomaterials hold great promise for medical, technological and economical benefits. Knowledge concerning the toxicological properties of these novel materials is typically lacking. At the same time, it is becoming evident that some nanomaterials could have a toxic potential in humans and the environment. Animal based systems lack the needed capacity to cope with the abundance of novel nanomaterials being produced, and thus we have to employ in vitro methods with high throughput to manage the rush logistically and use high content readouts wherever needed in order to gain more depth of information. Towards this end, high throughput screening (HTS) and high content screening (HCS) approaches can be used to speed up the safety analysis on a scale that commensurate with the rate of expansion of new materials and new properties. The insights gained from HTS/HCS should aid in our understanding of the tenets of nanomaterial hazard at biological level as well as assist the development of safe-by-design approaches. This review aims to provide a comprehensive introduction to the HTS/HCS methodology employed for safety assessment of engineered nanomaterials (ENMs), including data analysis and prediction of potentially hazardous material properties. Given the current pace of nanomaterial development, HTS/HCS is a potentially effective means of keeping up with the rapid progress in this field--we have literally no time to lose.
Figures
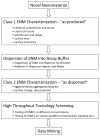
Assay Development Workflow for high throughput toxicity screening of Nanomaterials.
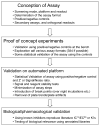
Assay Development Workflow. Adapted from: Molecular Screening R.Damoiseaux Handbook of Drug Discovery Wiley and Sons, forthcoming
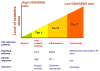
A mild oxidative stress activates the protective antioxidant machinery in an attempt to restore the redox equilibrium (Tier 1). Higher levels of oxidative stress activate MAPK and Nf-kB cascade of molecular events leading to pro-inflammatory responses (Tier 2). Oxidative stress beyond the tolerable limit of cells, triggers cell death pathways often manifested as increased cytosolic Ca2+ level, lowering of mitochondrial membrane potential, opening of mitochondrial permeability transition and cell membrane damage (Tier 3).
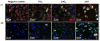
(i) BEAS-2B cells subjected to nanoparticles, stained with nucleic acid staining Hoechst 33342 and mitochondrial dye JC1. Healthy cells shows blue nuclei and red mitochondria (Negative control, TiO2 and CeO2) while the mitochondrial depolarization causes the cytoplasm to fluoresce green (green cells in ZnO). (ii) BEAS-2B cells treated with nanopaticles and stained with a dye cocktail of Hoechst 33342, fluo-4 (for intracellular Ca2+) and propidium iodide (for accessing membrane damage). Healthy cells shows blue nuclei, while damaged cells shows green cytoplasm and red nuclei (cells in ZnO group). Depending upon the color profile, the percentage of cells affected can be assayed and nanoparticles can be ranked for their cytotoxic potential.
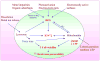
Nanomaterials induce ROS production as a direct consequence of specific material properties or as a consequence of triggering cellular injury responses leading to oxidant radical generation. ROS production could trigger a range of oxidative stress effects as outlined in the hierarchical oxidative stress model. The induction of cellular toxicity at the highest level of oxidative stress involves a number of interrelated cellular responses that include intracellular Ca2+ release and mitochondrial perturbation leading to cell death with accompanying changes in cell membrane integrity and nuclear PI uptake.
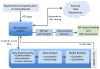
Components of a computational Framework for Predictive nano-Toxicology.
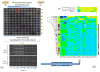
(a) Setup information for a HTS cytotoxicity assay showing plate arrangement. (b) Clustered heat-map corresponding to this data, blue colors indicate no activity, while yellow indicates toxic activity. Visual inspection identifies clusters of biologically active nanoparticles
Similar articles
-
Nel A, Xia T, Meng H, Wang X, Lin S, Ji Z, Zhang H. Nel A, et al. Acc Chem Res. 2013 Mar 19;46(3):607-21. doi: 10.1021/ar300022h. Epub 2012 Jun 7. Acc Chem Res. 2013. PMID: 22676423 Free PMC article. Review.
-
Holden PA, Nisbet RM, Lenihan HS, Miller RJ, Cherr GN, Schimel JP, Gardea-Torresdey JL. Holden PA, et al. Acc Chem Res. 2013 Mar 19;46(3):813-22. doi: 10.1021/ar300069t. Epub 2012 Oct 5. Acc Chem Res. 2013. PMID: 23039211
-
Liu Q, Wang X, Xia T. Liu Q, et al. Anal Bioanal Chem. 2018 Sep;410(24):6097-6111. doi: 10.1007/s00216-018-1289-y. Epub 2018 Aug 1. Anal Bioanal Chem. 2018. PMID: 30066194 Free PMC article. Review.
-
Nanomaterials in the environment: from materials to high-throughput screening to organisms.
Thomas CR, George S, Horst AM, Ji Z, Miller RJ, Peralta-Videa JR, Xia T, Pokhrel S, Mädler L, Gardea-Torresdey JL, Holden PA, Keller AA, Lenihan HS, Nel AE, Zink JI. Thomas CR, et al. ACS Nano. 2011 Jan 25;5(1):13-20. doi: 10.1021/nn1034857. ACS Nano. 2011. PMID: 21261306 Review.
-
In silico analysis of nanomaterials hazard and risk.
Cohen Y, Rallo R, Liu R, Liu HH. Cohen Y, et al. Acc Chem Res. 2013 Mar 19;46(3):802-12. doi: 10.1021/ar300049e. Epub 2012 Nov 8. Acc Chem Res. 2013. PMID: 23138971
Cited by
-
Nanomaterials toxicity and cell death modalities.
De Stefano D, Carnuccio R, Maiuri MC. De Stefano D, et al. J Drug Deliv. 2012;2012:167896. doi: 10.1155/2012/167896. Epub 2012 Dec 5. J Drug Deliv. 2012. PMID: 23304518 Free PMC article.
-
Kohl Y, Rundén-Pran E, Mariussen E, Hesler M, El Yamani N, Longhin EM, Dusinska M. Kohl Y, et al. Nanomaterials (Basel). 2020 Sep 25;10(10):1911. doi: 10.3390/nano10101911. Nanomaterials (Basel). 2020. PMID: 32992722 Free PMC article. Review.
-
Citrullination as early-stage indicator of cell response to single-walled carbon nanotubes.
Mohamed BM, Movia D, Knyazev A, Langevin D, Davies AM, Prina-Mello A, Volkov Y. Mohamed BM, et al. Sci Rep. 2013;3:1124. doi: 10.1038/srep01124. Epub 2013 Jan 24. Sci Rep. 2013. PMID: 23350031 Free PMC article.
-
Single nanoparticle detectors for biological applications.
Yurt A, Daaboul GG, Connor JH, Goldberg BB, Ünlü MS. Yurt A, et al. Nanoscale. 2012 Feb 7;4(3):715-26. doi: 10.1039/c2nr11562j. Epub 2012 Jan 3. Nanoscale. 2012. PMID: 22214976 Free PMC article. Review.
-
Labib S, Williams A, Yauk CL, Nikota JK, Wallin H, Vogel U, Halappanavar S. Labib S, et al. Part Fibre Toxicol. 2016 Mar 15;13:15. doi: 10.1186/s12989-016-0125-9. Part Fibre Toxicol. 2016. PMID: 26979667 Free PMC article.
References
-
- Baughman RH, Zakhidov AA, de Heer WA. Carbon nanotubes - the route toward applications. Science. 2002;297(5582):787–792. - PubMed
-
- Kotov NA, Winter JO, Clements IP, Jan E, Timko BP, Campidelli S, Pathak S, Mazzatenta A, Lieber CM, Prato M, Bellamkonda RV, Silva GA, Kam NWS, Patolsky F, Ballerini L. Nanomaterials for Neural Interfaces. Advanced Materials. 2009;21(40):3970–4004.
-
- Ariga K, Hu XL, Mandal S, Hill JP. By what means should nanoscaled materials be constructed: molecule, medium, or human? Nanoscale. 2010;2(2):198–214. - PubMed
-
- Chen J, Cheng FY. Combination of Lightweight Elements and Nanostructured Materials for Batteries. Accounts of Chemical Research. 2009;42(6):713–723. - PubMed
Publication types
MeSH terms
LinkOut - more resources
Full Text Sources
Other Literature Sources