Förster energy transfer theory as reflected in the structures of photosynthetic light-harvesting systems - PubMed
- ️Sat Jan 01 2011
Review
Förster energy transfer theory as reflected in the structures of photosynthetic light-harvesting systems
Melih Şener et al. Chemphyschem. 2011.
Abstract
Förster’s theory of resonant energy transfer underlies a fundamental process in nature, namely the harvesting of sunlight by photosynthetic life forms. The theoretical framework developed by Förster and others describes how electronic excitation migrates in the photosynthetic apparatus of plants, algae, and bacteria from light absorbing pigments to reaction centers where light energy is utilized for the eventual conversion into chemical energy. The demand for highest possible efficiency of light harvesting appears to have shaped the evolution of photosynthetic species from bacteria to plants which, despite a great variation in architecture, display common structural themes founded on the quantum physics of energy transfer as described first by Förster. Herein, Förster’s theory of excitation transfer is summarized, including recent extensions, and the relevance of the theory to photosynthetic systems as evolved in purple bacteria, cyanobacteria, and plants is demonstrated. Förster’s energy transfer formula, as used widely today in many fields of science, is also derived.
Copyright © 2011 WILEY-VCH Verlag GmbH & Co. KGaA, Weinheim.
Figures
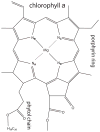
Structure of chlorophyll a molecule. The transition dipole moment of the lowest (Qy) state lies approximately in the plane of the porphyrin ring, along the vector connecting the NB and ND atoms.
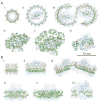
Pigment organization across different photosynthetic systems. (A) Top view (perpendicular to the membrane plane) and (B) side view (along the membrane plane) of pigment-protein complexes (i to vi) LH2 [42], RC-LH1 monomer [43], RC-LH1 dimer model [69], cyanobacterial PS1 [35], plant PS1 with Lhca subunits [37], and PS2 [38], respectively. Protein components are shown as transparent blue traces to highlight the Chls and BChls (green; shown only as porphyrin rings) and the carotenoids (orange). (See Supplementary Material for movies of these systems.)
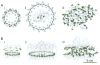
Orientations of the transition dipole moments of the constituent BChl and Chl molecules. (A) Top view (perpendicular to the plane of the membrane) and (B) side view (along the plane of the membrane); (i) LH2 [42], (ii) monomeric RC-LH1 [43, 147], and (iii) cyanobacterial PS1 [35].
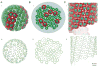
Structural models for (A) spherical [22, 23], (B) lamellar [121], and (C) tubular [55, 92] photosynthetic chromatophores from purple bacteria. Shown above are the constituent proteins: LH2 complexes (green) and RC-LH1 complexes (LH1 in red; RC in blue). Shown below are the associated BChl networks. The lamellar patch (B) is shown embedded in a lipid membrane, containing a total of nearly 23 million atoms including water (not shown). (See Supplementary Material for movies of these systems.)
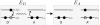
The excitation transfer process D*A → DA*. Here, φD and φA are the ground state orbitals of the donor and acceptor electrons and φD* and φA* are the excited state orbitals of the donor and acceptor electrons, respectively.
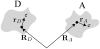
Positions of donor pigment RD and donor electron rD and of acceptor pigment RA and acceptor electron rA.
Similar articles
-
Schmitt FJ, Maksimov EG, Hätti P, Weißenborn J, Jeyasangar V, Razjivin AP, Paschenko VZ, Friedrich T, Renger G. Schmitt FJ, et al. Biochim Biophys Acta. 2012 Aug;1817(8):1461-70. doi: 10.1016/j.bbabio.2012.03.030. Epub 2012 Apr 4. Biochim Biophys Acta. 2012. PMID: 22503663
-
Photosynthetic Light-Harvesting (Antenna) Complexes-Structures and Functions.
Lokstein H, Renger G, Götze JP. Lokstein H, et al. Molecules. 2021 Jun 3;26(11):3378. doi: 10.3390/molecules26113378. Molecules. 2021. PMID: 34204994 Free PMC article. Review.
-
Fluorescence spectroscopy of single photosynthetic light-harvesting supramolecular systems.
Saga Y, Tamiaki H. Saga Y, et al. Cell Biochem Biophys. 2004;40(2):149-65. doi: 10.1385/CBB:40:2:149. Cell Biochem Biophys. 2004. PMID: 15054220 Review.
-
Atomistic study of energy funneling in the light-harvesting complex of green sulfur bacteria.
Huh J, Saikin SK, Brookes JC, Valleau S, Fujita T, Aspuru-Guzik A. Huh J, et al. J Am Chem Soc. 2014 Feb 5;136(5):2048-57. doi: 10.1021/ja412035q. Epub 2014 Jan 23. J Am Chem Soc. 2014. PMID: 24405318
-
Pachón LA, Brumer P. Pachón LA, et al. Phys Chem Chem Phys. 2012 Aug 7;14(29):10094-108. doi: 10.1039/c2cp40815e. Epub 2012 Jun 26. Phys Chem Chem Phys. 2012. PMID: 22735237 Review.
Cited by
-
Shedding Light on Primary Donors in Photosynthetic Reaction Centers.
Gorka M, Baldansuren A, Malnati A, Gruszecki E, Golbeck JH, Lakshmi KV. Gorka M, et al. Front Microbiol. 2021 Oct 1;12:735666. doi: 10.3389/fmicb.2021.735666. eCollection 2021. Front Microbiol. 2021. PMID: 34659164 Free PMC article. Review.
-
A synthetic biological quantum optical system.
Lishchuk A , Kodali G , Mancini JA , Broadbent M , Darroch B , Mass OA , Nabok A , Dutton PL , Hunter CN , Törmä P , Leggett GJ . Lishchuk A , et al. Nanoscale. 2018 Jul 13;10(27):13064-13073. doi: 10.1039/c8nr02144a. Nanoscale. 2018. PMID: 29956712 Free PMC article.
-
Ultrafast energy transfer between lipid-linked chromophores and plant light-harvesting complex II.
Hancock AM, Son M, Nairat M, Wei T, Jeuken LJC, Duffy CDP, Schlau-Cohen GS, Adams PG. Hancock AM, et al. Phys Chem Chem Phys. 2021 Sep 15;23(35):19511-19524. doi: 10.1039/d1cp01628h. Phys Chem Chem Phys. 2021. PMID: 34524278 Free PMC article.
-
Sponge-derived Ageladine A affects the in vivo fluorescence emission spectra of microalgae.
Peter C, Thoms S, Koch F, Sartoris FJ, Bickmeyer U. Peter C, et al. PLoS One. 2020 Nov 19;15(11):e0242464. doi: 10.1371/journal.pone.0242464. eCollection 2020. PLoS One. 2020. PMID: 33211752 Free PMC article.
-
Excited state dynamics in photosynthetic reaction center and light harvesting complex 1.
Strümpfer J, Schulten K. Strümpfer J, et al. J Chem Phys. 2012 Aug 14;137(6):065101. doi: 10.1063/1.4738953. J Chem Phys. 2012. PMID: 22897312 Free PMC article.
References
-
- van Amerongen H, Valkunas L, van Grondelle R. World Scientific; Singapore: 2000.
-
- Blankenship RE. Molecular Mechanisms of Photosynthesis. Blackwell Science; Malden, MA: 2002.
-
- Cogdell RJ, Gall A, Köhler J. Quart Rev Biophys. 2006;39:227–324. - PubMed
-
- Şener MK, Schulten K. In: The Purple Phototrophic Bacteria, volume 28 of Advances in Photosynthesis and Respiration. Hunter CN, Daldal Fevzi, Thurnauer Marion C, Thomas Beatty J, editors. Springer; 2008. pp. 275–294.
-
- Förster T. Naturwissenschaften. 1946;6:166–175.
Publication types
MeSH terms
Substances
Grants and funding
LinkOut - more resources
Full Text Sources