Formyltetrahydrofolate synthetase gene diversity in the guts of higher termites with different diets and lifestyles - PubMed
Formyltetrahydrofolate synthetase gene diversity in the guts of higher termites with different diets and lifestyles
Elizabeth A Ottesen et al. Appl Environ Microbiol. 2011 May.
Abstract
In this study, we examine gene diversity for formyl-tetrahydrofolate synthetase (FTHFS), a key enzyme in homoacetogenesis, recovered from the gut microbiota of six species of higher termites. The "higher" termites (family Termitidae), which represent the majority of extant termite species and genera, engage in a broader diversity of feeding and nesting styles than the "lower" termites. Previous studies of termite gut homoacetogenesis have focused on wood-feeding lower termites, from which the preponderance of FTHFS sequences recovered were related to those from acetogenic treponemes. While sequences belonging to this group were present in the guts of all six higher termites examined, treponeme-like FTHFS sequences represented the majority of recovered sequences in only two species (a wood-feeding Nasutitermes sp. and a palm-feeding Microcerotermes sp.). The remaining four termite species analyzed (a Gnathamitermes sp. and two Amitermes spp. that were recovered from subterranean nests with indeterminate feeding strategies and a litter-feeding Rhynchotermes sp.) yielded novel FTHFS clades not observed in lower termites. These termites yielded two distinct clusters of probable purinolytic Firmicutes and a large group of potential homoacetogens related to sequences previously recovered from the guts of omnivorous cockroaches. These findings suggest that the gut environments of different higher termite species may select for different groups of homoacetogens, with some species hosting treponeme-dominated homoacetogen populations similar to those of wood-feeding, lower termites while others host Firmicutes-dominated communities more similar to those of omnivorous cockroaches.
Figures
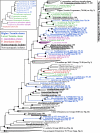
Phylogenetic analysis of higher termite FTHFS clones and selected relatives. The tree was constructed using 317 unambiguous, aligned amino acids and the PhyML maximum-likelihood algorithm. Homoacetogen similarity (HS) scores are shown for each isolate or environmental clone. Open circles indicate nodes also supported by either the Fitch distance or Phylip parsimony method. Closed circles indicate nodes supported by all three algorithms. The scale bar indicates 0.1 changes per alignment position. Inset: phylogenetic analysis of termite and roach mitochondrial COII genes. The tree was calculated using 394 unambiguous, aligned DNA bases. The scale bar indicates 0.1 changes per alignment position. A more detailed phylogenetic analysis of COII genes is presented in Fig. S1 in the supplemental material.
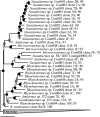
Phylogenetic analysis of the higher termite treponeme clade. Tree were constructed using 344 unambiguous, aligned amino acids and the PhyML maximum-likelihood algorithm. The HS score is shown next to each clone name. Open circles indicate nodes also supported by either the Fitch distance or Phylip parsimony method. Closed circles indicate nodes supported by all three algorithms. The scale bar indicates 0.1 changes per alignment position. The outgroup consisted of the 13 acetogenic isolates in Fig. 1 plus Treponema spp. ZAS-8 and ZAS-9.
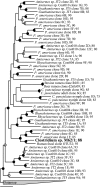
Phylogenetic analysis of the Clostridium sp. M62/1 clade. The tree was constructed using 327 unambiguous, aligned amino acids and the PhyML maximum-likelihood algorithm. The HS score is shown next to each clone. Open circles indicate nodes also supported by either the Fitch distance or Phylip parsimony method. Closed circles indicate nodes supported by all three algorithms. The scale bar indicates 0.1 changes per alignment position. The 13 acetogenic isolates in Fig. 1 were used as an outgroup.
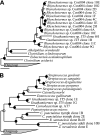
Phylogenetic analysis of the Clostridium acidurici (A) and clone E/Streptococcus (B) clades. Trees were constructed using 344 (C. acidurici) or 343 (clone E) unambiguous, aligned amino acids and the PhyML maximum-likelihood algorithm. Open circles indicate nodes also supported by either the Fitch distance or Phylip parsimony method. Closed circles indicate nodes supported by all three algorithms. Scale bars indicate 0.1 changes per alignment position. The 13 acetogenic isolates in Fig. 1 were used as an outgroup for both trees.
Similar articles
-
Ottesen EA, Leadbetter JR. Ottesen EA, et al. Appl Environ Microbiol. 2010 Jul;76(14):4909-13. doi: 10.1128/AEM.00299-10. Epub 2010 May 21. Appl Environ Microbiol. 2010. PMID: 20495046 Free PMC article.
-
Salmassi TM, Leadbetter JR. Salmassi TM, et al. Microbiology (Reading). 2003 Sep;149(Pt 9):2529-2537. doi: 10.1099/mic.0.26351-0. Microbiology (Reading). 2003. PMID: 12949177
-
Ballor NR, Leadbetter JR. Ballor NR, et al. Appl Environ Microbiol. 2012 Aug;78(15):5368-74. doi: 10.1128/AEM.08008-11. Epub 2012 May 25. Appl Environ Microbiol. 2012. PMID: 22636002 Free PMC article.
-
[Diversity and function of symbiotic microbes in the gut of lower termites].
Yang H, Peng JX, Liu KY, Hong HZ. Yang H, et al. Wei Sheng Wu Xue Bao. 2006 Jun;46(3):496-9. Wei Sheng Wu Xue Bao. 2006. PMID: 16933630 Review. Chinese.
-
Challenges and physiological implications of wood feeding in termites.
Scharf ME. Scharf ME. Curr Opin Insect Sci. 2020 Oct;41:79-85. doi: 10.1016/j.cois.2020.07.007. Epub 2020 Aug 8. Curr Opin Insect Sci. 2020. PMID: 32823202 Review.
Cited by
-
He S, Ivanova N, Kirton E, Allgaier M, Bergin C, Scheffrahn RH, Kyrpides NC, Warnecke F, Tringe SG, Hugenholtz P. He S, et al. PLoS One. 2013 Apr 12;8(4):e61126. doi: 10.1371/journal.pone.0061126. Print 2013. PLoS One. 2013. PMID: 23593407 Free PMC article.
-
Mi L, Yang B, Hu X, Luo Y, Liu J, Yu Z, Wang J. Mi L, et al. Front Microbiol. 2018 Mar 27;9:575. doi: 10.3389/fmicb.2018.00575. eCollection 2018. Front Microbiol. 2018. PMID: 29662480 Free PMC article.
-
Loh HQ, Hervé V, Brune A. Loh HQ, et al. Front Microbiol. 2021 Feb 3;11:635786. doi: 10.3389/fmicb.2020.635786. eCollection 2020. Front Microbiol. 2021. PMID: 33613473 Free PMC article.
-
Zhang X, Leadbetter JR. Zhang X, et al. mBio. 2012 Aug 21;3(4):e00223-12. doi: 10.1128/mBio.00223-12. Print 2012. mBio. 2012. PMID: 22911968 Free PMC article.
-
Hervé V, Liu P, Dietrich C, Sillam-Dussès D, Stiblik P, Šobotník J, Brune A. Hervé V, et al. PeerJ. 2020 Feb 13;8:e8614. doi: 10.7717/peerj.8614. eCollection 2020. PeerJ. 2020. PMID: 32095380 Free PMC article.
References
-
- Bitsch C., Noirot C. 2002. Gut characters and phylogeny of the higher termites (Isoptera: Termitidae). A cladistic analysis. Ann. Soc. Entomol. Fr. 38:201–210
-
- Brauman A., Kane M. D., Labat M., Breznak J. A. 1992. Genesis of acetate and methane by gut bacteria of nutritionally diverse termites. Science 257:1384–1387 - PubMed
-
- Engel M. S., Krishna K. 2004. Family-group names for termites (Isoptera). Am. Mus. Novit. 3432:1–9 - PubMed
Publication types
MeSH terms
Substances
Grants and funding
LinkOut - more resources
Full Text Sources
Miscellaneous