PCSK9 reduces the protein levels of the LDL receptor in mouse brain during development and after ischemic stroke - PubMed
PCSK9 reduces the protein levels of the LDL receptor in mouse brain during development and after ischemic stroke
Estelle Rousselet et al. J Lipid Res. 2011 Jul.
Abstract
Proprotein convertase subtilisin/kexin type 9 (PCSK9) plays a major role in cholesterol homeostasis through enhanced degradation of the LDL receptor (LDLR) in liver. As novel inhibitors/silencers of PCSK9 are now being tested in clinical trials to treat hypercholesterolemia, it is crucial to define the physiological consequences of the lack of PCSK9 in various organs. LDLR regulation by PCSK9 has not been extensively described during mouse brain development and injury. Herein, we show that PCSK9 and LDLR are co-expressed in mouse brain during development and at adulthood. Although the protein levels of LDLR and apolipoprotein E (apoE) in the adult brain of Pcsk9(-/-) mice are similar to those of wild-type (WT) mice, LDLR levels increased and were accompanied by a reduction of apoE levels during development. This suggests that the upregulation of LDLR protein levels in Pcsk9(-/-) mice enhances apoE degradation. Upon ischemic stroke, PCSK9 was expressed in the dentate gyrus between 24 h and 72 h following brain reperfusion. Although mouse behavior and lesion volume were similar, LDLR protein levels dropped ∼2-fold less in the Pcsk9(-/-)-lesioned hippocampus, without affecting apoE levels and neurogenesis. Thus, PCSK9 downregulates LDLR levels during brain development and following transient ischemic stroke in adult mice.
Figures
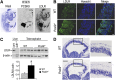
LDLR regulation by PCSK9 in mouse telencephalon at E12.5. A: PCSK9 and LDLR mRNA distribution pattern in cryosections of sagital mouse embryo at E12.5 by in situ hybridization using PCSK9 and LDLR antisense riboprobes and followed by Nissl staining. FCx, frontal cortex; Int, small intestine. B: Immunofluorescence of LDLR on cryosections of E12.5 WT and Pcsk9−/− mice. Nuclei were stained by Hoescht 33258. Bar = 30 μm. C: Representative immunoblots showing LDLR and β-actin protein levels in the telencephalon at E12.5 (100 μg protein load/lane) and in adult liver (30 μg protein load/lane) of WT and Pcsk9−/− mice. The specificity of the LDLR antibody is emphasized by the absence of signal in the liver of Ldlr−/− mice. Bar diagrams represent the protein levels of LDLR normalized to those of β-actin (n = 10 for WT mice and n = 13 for Pcsk9−/− mice). *P < 0.05 by Student-Newman-Keuls. All error bars represent SEM. D: Nissl staining showing the cell layer organization in the telencephalon of WT and Pcsk9−/− mice. The enlarged squared regions are shown on the right. Bars = 200 μm (left) and 40 μm (right).
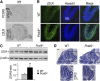
LDLR regulation by PCSK9 in mouse brain at P7. A: In situ hybridization of sagital mouse brain cryosections at P7 with a PCSK9 and LDLR antisense 35S-labeled cRNA riboprobes. B: Immunofluorescence of LDLR in cerebellum cryosections at P7 from WT and Pcsk9−/− mice. Nuclei were stained by Hoescht 33258. Bar = 20 μm. C: Representative immunoblots showing LDLR and β-actin protein levels (100 μg protein load/lane) in the cerebellum of WT and Pcsk9−/− mice at P7. Bar diagrams represent the LDLR protein levels normalized to those of β-actin (n = 5 for WT and Pcsk9−/− mice). *P < 0.05 by Student-Newman-Keuls. All error bars represent the SEM. D: Nissl staining showing the cell layer organization in the cerebellum of WT and Pcsk9−/− mice at P7. The enlarged squared regions are shown below. Bars = 250 μm (upper) and 150 μm (lower).
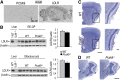
LDLR regulation by PCSK9 at adulthood. A: In situ hybridization of sagital adult mouse brain cryosections with a PCSK9 and LDLR antisense 35S-labeled cRNA riboprobes. B: Representative immunoblots showing LDLR and β-actin protein levels in the RE-OP and olfactory bulb (200 μg protein load/lane) and in adult liver (30 μg of protein load/lane) of WT and Pcsk9−/− mice. The specificity of the LDLR antibody is emphasized by the absence of signal in the liver of Ldlr−/− mice. Bar diagrams represent the protein levels of LDLR normalized to those of β-actin in RE-OP and olfactory bulb (n = 9 for WT and Pcsk9−/− mice). All error bars represent the SEM. C: Nissl staining showing the structure of the RE-OP in WT and Pcsk9−/− mice. The enlarged squared regions are shown on the right. Bars = 400 μm (left) and 100 μm (right). D: Nissl staining revealing the structure of the olfactory bulb in WT and Pcsk9−/− mice. Bar = 1 mm.
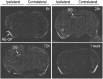
Time-dependent PCSK9 expression following an ischemic stroke. mRNA distribution pattern using PCSK9 antisense riboprobe, seen as bright labeling under darkfield illumination in mouse coronal cryosections following transient (1 h) tMCAO at different times following brain reperfusion (6, 24, and 72 h and 1 week). DG, hippocampal dentate gyrus .
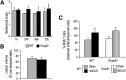
Impact of ischemic stroke on Pcsk9−/− mice. A: Behavior analysis after a tMCAO (1 h) ischemic stroke at different times of brain reperfusion (1, 24, 48, and 72 h) of WT and Pcsk9−/− mice. B: Lesion volume at 72 h of brain reperfusion following tMCAO in WT and Pcsk9−/− mice. C: BrdU was injected intraperitoneally for two days (twice a day at 8 h intervals) following tMCAO versus in sham-operated mice. WT and Pcsk9−/− mice were euthanized at 72 h of brain reperfusion. BrdU positive cells were counted in ipsilateral (lesioned side) and contralateral (nonlesioned side) dentate gyrus. Bar diagrams represent the percent ratio of the number of BrdU positive cells in the ipsilateral side versus the contralateral one (n = 5 for WT and Pcsk9−/− mice). All error bars represent the SEM.
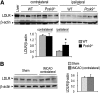
LDLR regulation by PCSK9 following tMCAO. A: Representative immunoblots showing the protein levels of LDLR and β-actin in the hippocampus of WT and Pcsk9−/− mice following tMCAO (1 h) and 24 h of brain reperfusion (200 μg protein load/lane) and in adult liver (30 μg protein load/lane). Ipsilateral and contralateral hippocampi were isolated and analyzed separately. Bar diagrams represent the quantitation of the protein levels of LDLR normalized to those of β-actin (n = 4 for WT and Pcsk9−/− mice). *P < 0.05 by Dunnett test. All error bars represent the SEM. B: Representative immunoblots showing the protein levels of LDLR and β-actin in the contralateral hippocampus of WT mice following or not tMCAO (1 h) and 24 h of brain reperfusion (200 μg protein load/lane). Bar diagrams represent the quantitation of the protein levels of LDLR normalized to those of β-actin (n = 4 for sham and n = 3 for tMCAO).
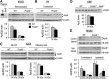
Regulation of apoE protein levels in Pcsk9−/− mouse brain during development, at adulthood, and following tMCAO. Representative immunoblots (100 μg protein load/lane) of WT and Pcsk9−/− mice showing the protein levels of apoE and β-actin in the telencephalon at E12.5 (A), in the cerebellum at P7 (B), at adulthood in the REOP and olfactory bulb (C), in adult CSF (D), and in the adult contralateral and ipsilateral hippocampus following tMCAO (E). The specificity of the apoE antibody is emphasized by the absence of signal in the plasma and CSF of apoE−/− mice (A, D). Bar diagrams represent the normalized protein levels of apoE to those of β-actin (n = 4). The error bars represent the SEM.
Similar articles
-
Ason B, van der Hoorn JW, Chan J, Lee E, Pieterman EJ, Nguyen KK, Di M, Shetterly S, Tang J, Yeh WC, Schwarz M, Jukema JW, Scott R, Wasserman SM, Princen HM, Jackson S. Ason B, et al. J Lipid Res. 2014 Nov;55(11):2370-9. doi: 10.1194/jlr.M053207. Epub 2014 Sep 25. J Lipid Res. 2014. PMID: 25258384 Free PMC article.
-
Miranda MX, van Tits LJ, Lohmann C, Arsiwala T, Winnik S, Tailleux A, Stein S, Gomes AP, Suri V, Ellis JL, Lutz TA, Hottiger MO, Sinclair DA, Auwerx J, Schoonjans K, Staels B, Lüscher TF, Matter CM. Miranda MX, et al. Eur Heart J. 2015 Jan 1;36(1):51-9. doi: 10.1093/eurheartj/ehu095. Epub 2014 Mar 6. Eur Heart J. 2015. PMID: 24603306 Free PMC article.
-
Proprotein convertase subtilisin/kexin type 9 deficiency reduces melanoma metastasis in liver.
Sun X, Essalmani R, Day R, Khatib AM, Seidah NG, Prat A. Sun X, et al. Neoplasia. 2012 Dec;14(12):1122-31. doi: 10.1593/neo.121252. Neoplasia. 2012. PMID: 23308045 Free PMC article.
-
Urban D, Pöss J, Böhm M, Laufs U. Urban D, et al. J Am Coll Cardiol. 2013 Oct 15;62(16):1401-8. doi: 10.1016/j.jacc.2013.07.056. Epub 2013 Aug 21. J Am Coll Cardiol. 2013. PMID: 23973703 Review.
-
The unique role of proprotein convertase subtilisin/kexin 9 in cholesterol homeostasis.
Mousavi SA, Berge KE, Leren TP. Mousavi SA, et al. J Intern Med. 2009 Dec;266(6):507-19. doi: 10.1111/j.1365-2796.2009.02167.x. J Intern Med. 2009. PMID: 19930098 Review.
Cited by
-
Mouse model of intraluminal MCAO: cerebral infarct evaluation by cresyl violet staining.
Rousselet E, Kriz J, Seidah NG. Rousselet E, et al. J Vis Exp. 2012 Nov 6;(69):4038. doi: 10.3791/4038. J Vis Exp. 2012. PMID: 23168377 Free PMC article.
-
Hypercholesterolemia and the Increased Risk of Vascular Dementia: a Cholesterol Perspective.
Shang G, Shao Q, Lv K, Xu W, Ji J, Fan S, Kang X, Cheng F, Wang X, Wang Q. Shang G, et al. Curr Atheroscler Rep. 2024 Aug;26(8):435-449. doi: 10.1007/s11883-024-01217-3. Epub 2024 May 30. Curr Atheroscler Rep. 2024. PMID: 38814418 Review.
-
A bibliometric analysis of PCSK9 inhibitors from 2007 to 2022.
Luo Q, Tang Z, Wu P, Chen Z, Fang Z, Luo F. Luo Q, et al. Front Endocrinol (Lausanne). 2023 Nov 29;14:1218968. doi: 10.3389/fendo.2023.1218968. eCollection 2023. Front Endocrinol (Lausanne). 2023. PMID: 38093957 Free PMC article.
-
Swiger KJ, Martin SS. Swiger KJ, et al. Drug Saf. 2015 Jun;38(6):519-26. doi: 10.1007/s40264-015-0296-6. Drug Saf. 2015. PMID: 25989944
-
Picard C, Poirier A, Bélanger S, Labonté A, Auld D, Poirier J; PREVENT-AD Research Group. Picard C, et al. PLoS One. 2019 Aug 22;14(8):e0220254. doi: 10.1371/journal.pone.0220254. eCollection 2019. PLoS One. 2019. PMID: 31437157 Free PMC article.
References
-
- Seidah N. G., Benjannet S., Wickham L., Marcinkiewicz J., Jasmin S. B., Stifani S., Basak A., Prat A., Chretien M. 2003. The secretory proprotein convertase neural apoptosis-regulated convertase 1 (NARC-1): liver regeneration and neuronal differentiation. Proc. Natl. Acad. Sci. USA. 100: 928–933. - PMC - PubMed
-
- Abifadel M., Varret M., Rabes J. P., Allard D., Ouguerram K., Devillers M., Cruaud C., Benjannet S., Wickham L., Erlich D., et al. 2003. Mutations in PCSK9 cause autosomal dominant hypercholesterolemia. Nat. Genet. 34: 154–156. - PubMed
-
- Allard D., Amsellem S., Abifadel M., Trillard M., Devillers M., Luc G., Krempf M., Reznik Y., Girardet J. P., Fredenrich A., et al. 2005. Novel mutations of the PCSK9 gene cause variable phenotype of autosomal dominant hypercholesterolemia. Hum. Mutat. 26: 497–506. - PubMed
Publication types
MeSH terms
Substances
LinkOut - more resources
Full Text Sources
Molecular Biology Databases
Research Materials
Miscellaneous