The molecular physiology of CRH neurons - PubMed
The molecular physiology of CRH neurons
Greti Aguilera et al. Front Neuroendocrinol. 2012 Jan.
Abstract
Corticotropin releasing hormone (CRH) is essential for stress adaptation by mediating hypothalamic-pituitary-adrenal (HPA) axis, behavioral and autonomic responses to stress. Activation of CRH neurons depends on neural afferents from the brain stem and limbic system, leading to sequential CRH release and synthesis. CRH transcription is required to restore mRNA and peptide levels, but termination of the response is essential to prevent pathology associated with chronic elevations of CRH and HPA axis activity. Inhibitory feedback mediated by glucocorticoids and intracellular production of the repressor, Inducible Cyclic AMP Early Repressor (ICER), limit the magnitude and duration of CRH neuronal activation. Induction of CRH transcription is mediated by the cyclic AMP/protein kinase A/cyclic AMP responsive element binding protein (CREB)-dependent pathways, and requires cyclic AMP-dependent nuclear translocation of the CREB co-activator, Transducer of Regulated CREB activity (TORC). This article reviews current knowledge on the mechanisms regulating CRH neuron activity.
Copyright © 2011. Published by Elsevier Inc.
Figures
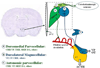
In situ hybridization of CRH mRNA in a coronal section of rat brain at the level of the hypothalamus showing autoradiographic staining in the dorsomedial and autonomic parvocellular subdivisions. A diagram of the different subdivisions of the region indicated by the dotted lines square is shown on the right. (a) The dorsomedial parvocellular division contains corticotrophin releasing hormone (CRH) neurons, about 50% co-expressing vasopressin (VP). These neurons project to the external zone of the median eminence and are responsible for the stimulation of ACTH release and synthesis by corticotrophs in the anterior pituitary lobe (apl). This region also contains somatostatin (SRIF), thyrotropin releasing hormone (TRH), dopaminergic (DA), growth hormone releasing hormone (GRF) and other peptidergic neurons including enkephalins, dynorphin, galanin, cholecystokinin, angiotensin and neurotensin. (b) The dorsolateral magnocellular subdivision containing VP and oxytocin neurons, projecting to the posterior pituitary lobe (ppl), and responsible for the release of OT and VP to the peripheral circulation. Oxytocinergic neurons co-express small amounts of CRH, which increases during stress and osmotic stimulation. Magnocellular neurons also express dynophin and cholecystokinin. (c) The autonomic parvocellular neurons, containing CRH, and small quantities of most peptides found in the dorsomedial and dorsolateral subdivisions.
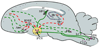
CRH neurons depicted as purple dots are mainly concentrated in the PVN but also present in catecholaminergic neurons of the brain stem, frontal cortex, central nucleus of the amygdala (c), bed nucleus of the stria terminalis (BNST) and other limbic areas. Systemic stressors (blood loss, pain, hypoglycemia) signal through direct (solid green lines) projections to the CRH neuron from A2 and C2 catecholaminergic neurons in the nucleus of the solitary tract (NTS), and A1 and C1 adrenergic neurons in the ventrolateral medulla. Signals from psychogenic stressors utilize multisynaptic pathways from sensory organs, the locus coeruleus (LC) and brain stem nuclei through limbic structures, including the prefrontal cortex, bed nucleus of the stria terminalis (BNST), and amygdala. This pathways interact with the CRH neuron indirectly (doted lines) through glutamatergic and GABAergic neurons in the peri-PVN area (p-p), which make direct synapses with the CRH neuron. The hippocampus sends inhibitory signals through the prefrontal cortex and subiculum (Sbc). The dorsal raphe projects serotoninergic signals to the peri-PVN area and directly to the CRH neuron. In addition, hormonal signals from the periphery interact with the subfornical organ (SFO), a circumventricular organ outside the blood brain barrier, which has direct projections to the CRH neuron.
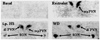
In situ hybridization images for CRHR1 mRNA in hypothalamic sections of control rats, 4h after 1h restraint stress, or i.p. hypertonic saline injection (ipHS), or osmotic stimulation by 60 h water deprivation (WD). Levels of CRHR1 mRNA in the PVN are very low in basal conditions and increased markedly in the dorsomedial parvocellular region of the PVN (corresponding to CRH neurons) after a psychosomatic stress (restraint) and in the dorsolateral magnocellular PVN and SON following osmotic stimulation (WD). Hypertonic saline injection (i.p.), a combined painful stress and osmotic stimulation induces CRHR1 mRNA in both parvocellular and magnocellular neurons.
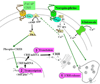
Stimulation of afferent neural pathways to the PVN results in the release of norepinephrine and glutamate. Norepinephrine acts on alpha adrenergic receptors which are coupled to the guanyl nucleotide binding protein q/11 (Gq11) and phospolipase C (PLC), resulting in increase in intracellular calcium (Ca2+) and protein kinase C (PKC) activity. Glutamate interacts with NMDA and GluR5 receptors leading to increases in intracellular calcium. These pathways are likely to mediate rapid CRH release within seconds . Release is follow by activation of CRH transcription within minutes
, a process which requires cyclic AMP. The mechanism for cyclic AMP production is not clear but it could involve activation of CRHR1 or PACAP receptors, which are coupled to adenylate cyclase, or/and activation of a calcium dependent adenylate cyclase (indicated by the broken arrow). Transcription leads to increases in mRNA levels and translation to the CRH precursor protein
. The signaling mechanisms controlling translation and processing of the precursor protein to CRH have not been fully elucidated.
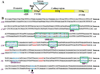
As shown in A, the CRH gene has two exons (shown in yellow) and one intron (shown in blue) located in the 5’ untranslated region (5’UTR). The 5’ flanking region is shown in green and emphasizes the cyclic AMP response element (CRE) at −229, which is essential for transcriptional activation and inhibition. A comparison of the sequences and response elements in the 5’ flanking region showing the high homology between human and rat is depicted in B. In both species the promoter has 2 TATA boxes or TATA binding protein elements (TBP), the CRE at −229, a combined glucocorticoid and AP1 response element (GRE/AP1), and an a half estrogen response element (ERE). These elements have been characterized using reporter gene assays. In addition, there are several potential sites for the POU homeodomain protein, Brn-2, which is essential for CRH neurons differentiation (indicated in red), Several other motifs present in the promoter have not been functionally characterized.
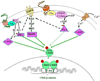
Activation of G protein coupled receptors, receptor and voltage activated calcium channels shown in Fig 4, leads to increases in intracellular calcium, and other second messengers such as inositol 3 phosphate (IP3), diacylglycerol (DG) and cyclic AMP (cAMP) and consequent activation of calcium calmodulin dependent protein kinase (CaMK), protein kinase C (PKC), protein kinase A (PKA) and transactivation of the mitogen activated protein kinase (MAPK). All of these protein kinases can activate Cyclic AMP Responsive Element Binding protein (CREB), by phosphorylation. However, CREB phosphorylation alone is not sufficient to initiate CRH transcription.
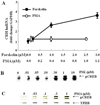
The dose responses for the effect of the stimulator of adenylate cyclase, forskolin and the phorbol ester, PMA, on CRH hnRNA in primary cultures of hypothalamic neurons show dose dependent increases after incubation for 45 min with forskolin but not after incubation with PMA. CRH hnRNA was measured by qRT-PCR using intronic primers (A). Western blot analyses of phospho-CREB content in nuclear proteins hypothalamic cells, 4B, incubated for 30 min with forskolin (B) or PMA (C).
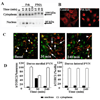
Time course of the effect of forskolin and PMA on nuclear translocation of TORC2 in 4B cells Cytoplasmic and nuclear proteins from cells treated with vehicle (0.01% DMSO 0), forskolin (Fsk), or PMA were subjected to Western blot analysis for TORC2. Data points are the mean ± SE of the values obtained in three experiments, expressed as fold-change from basal values in vehicle treated cells (A). Immunofluorecence staining of TORC 2 in 4B cells treated for 30 min with vehicle or forskolin (B). Co-localization of TORC 2 and CRH in parvocellular neurons of the hypothalamic sections of control rats, and in rats subjected to restraint stress for 30 min or 180 min after 1h restraint (240 min) (C). The quantitative analysis of the number of cells showing nuclear and cytoplasmic staining in the dorsomedial (parvocellular) and dorsolateral (magnocellular) regions of the PVN is shown in (D). Bars represent the mean and SE of measurements expressed as percent of cytosolic and nuclear location per total number of cells were counted in each animal in three rats. *P < 0.01 higher than nuclear staining in basal (time 0), or 3 h after termination of 1 h of stress (240 min). #P < 0.01 lower than cytoplasmic staining in basal, or 3 h after termination of 1 h of stress (240 min).
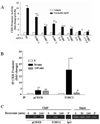
(A) Silencing (si)RNA blockade of specific TORC subtypes reduces forkolin-stimulated CRH transcription in primary cultures of hypothalamic neurons. Neuronal cultures were transfected with a non specific (NS), or TORC1, TORC2 or TORC 3 siRNA, and 24h later incubated for 45min with forskolin (Fsk) or vehicle (0.01% DMSO) before preparation of total RNA for determination of TORC mRNA and CRH hnRNA by qRT-PCR. Bars are the mean and SE of data obtained in 4 experiments, expressed as fold-change from vehicle in cells transfected with NS. **, p<0.001 siRNA vs. NS (TORC mRNA), or forskolin vs. respective vehicle; *, or p<0.03 forskolin vs. respective vehicle; #, p< 0.001 forskolin siRNA vs.forskolin non-specific oligonucleotide. (B) Restraint stress induces recruitment of phospho-CREB and TORC2 by the CRH promoter in the hypothamic PVN region. ChIP assays using phospho-CREB and TORC 2 antibodies and cross-linked DNA from microdissected hypothalamic PVN region of control rats and rats subjected to restraint stress for 30min or 3h after 1h restraint (240 min) (A). Amounts of CRH promoter DNA in the immunoprecipitant were measured by qRT-PCR. Bars represent the mean and SE of the results obtained in 4 experiments using pooled tissue from 2 or 3 rats per experimental point. (C) Representative gel showing the results obtained in pools of 3 hypothalamic sections per group in a representative experiment (B). **, p< 0.01 higher than basal; **, p<0.02 higher than basal; #, p<0.02 lower than 30 min.
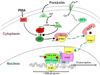
In basal conditions TORC is in the cytoplasm, in an inactive state phosphorylated at Ser 171 and bound to the scaffolding protein 14-3-3. Based on the described effect of the phorbol ester, PMA, on TORC phosphorylation, and known ability of salt inducible kinase (SIK) to phosphorylate TORC, it is likely that protein kinase C (PKC) activates SIK, which in turn maintains TORC phosphorylated in the cytoplasm. Stimulation of adenylate cyclase (AC) by forskolin or a G-protein coupled receptor (not shown) leads to cyclic AMP production and stimulation of protein kinase A (PKA), which phosphorylates SIK at Ser 577 and inactivates it, blocking TORC phosphorylation. In addition, increase in intracellular calcium stimulates the phosphatase, calcineurin (PPP3), which has been shown to dephosphorylate TORC. Upon dephosphorylation, TORC dissociates from 14-3-3 nad translocates to the nucleus (indicated by the green dotted lines) where it interacts with CREB at the cyclic AMP response element in the CRH promoter and facilitates the recruitment the transcription initiation complex including CREB binding protein (CBP)/p300, TATA binding protein (TBP), TBP associated factors (TAFII) and RNA polymerase II (Pol II).
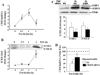
Time course of the effects of forskolin on ICER I and II production (A) and CRH transcription (B) Cell were incubated for the time periods indicated before processing for western blot analysis or CRH hnRNA determination by intronic real time PCR (B). Data points are the mean and SE of the values in 3 experiments. *, p<0.001 vs time 0; #, p<0.001 vs 1h. Blockade of endogenous ICER in primary cultures of hypothalamic neurons using CREM siRNA reduced forskolin-induced ICER I and II protein levels (C) and CRH hnRNA levels (D). Cells were incubated for 3h with forskolin (Fsk) before preparation of protein extracts for western blot. Bars are the mean and SE of ICER, expressed as percent of forskolin-stimulated values in cells transfected with the non-specific oligonucleotide *, p<0.05. CRH hnRNA levels are expressed as percent of stimulation at 1h. As shown in D, CREM siRNA significantly reduced the decline in CRH hnRNA observed after 3h incubation with forskolin. *, p<0.05 CREM siRNA compared with non-specific oligonucleotide (NS).
Similar articles
-
Negative regulation of corticotropin releasing factor expression and limitation of stress response.
Aguilera G, Kiss A, Liu Y, Kamitakahara A. Aguilera G, et al. Stress. 2007 Jun;10(2):153-61. doi: 10.1080/10253890701391192. Stress. 2007. PMID: 17514584
-
Légrádi G, Holzer D, Kapcala LP, Lechan RM. Légrádi G, et al. Neuroendocrinology. 1997 Aug;66(2):86-97. doi: 10.1159/000127224. Neuroendocrinology. 1997. PMID: 9263205
-
Liu Y, Poon V, Sanchez-Watts G, Watts AG, Takemori H, Aguilera G. Liu Y, et al. Endocrinology. 2012 Jan;153(1):223-33. doi: 10.1210/en.2011-1404. Epub 2011 Nov 22. Endocrinology. 2012. PMID: 22109884 Free PMC article.
-
CRH: the link between hormonal-, metabolic- and behavioral responses to stress.
Kovács KJ. Kovács KJ. J Chem Neuroanat. 2013 Dec;54:25-33. doi: 10.1016/j.jchemneu.2013.05.003. Epub 2013 Jun 15. J Chem Neuroanat. 2013. PMID: 23774011 Review.
-
Regulatory mechanisms underlying corticotropin-releasing factor gene expression in the hypothalamus.
Kageyama K, Suda T. Kageyama K, et al. Endocr J. 2009;56(3):335-44. doi: 10.1507/endocrj.k09e-075. Epub 2009 Apr 7. Endocr J. 2009. PMID: 19352056 Review.
Cited by
-
Ma S, Blasiak A, Olucha-Bordonau FE, Verberne AJ, Gundlach AL. Ma S, et al. J Physiol. 2013 Aug 15;591(16):3981-4001. doi: 10.1113/jphysiol.2013.254300. Epub 2013 May 13. J Physiol. 2013. PMID: 23671163 Free PMC article.
-
Differentiation, Transcriptomic Profiling, and Calcium Imaging of Human Hypothalamic Neurons.
Chen HC, Mazzaferro S, Tian T, Mali I, Merkle FT. Chen HC, et al. Curr Protoc. 2023 Jun;3(6):e786. doi: 10.1002/cpz1.786. Curr Protoc. 2023. PMID: 37272700 Free PMC article.
-
Zhang H, Zhou JJ, Shao JY, Sheng ZF, Wang J, Zheng P, Kang X, Liu Z, Cheng ZJ, Kline DD, Li DP. Zhang H, et al. J Neurosci. 2023 Jun 14;43(24):4513-4524. doi: 10.1523/JNEUROSCI.2343-22.2023. Epub 2023 May 9. J Neurosci. 2023. PMID: 37160364 Free PMC article.
-
Palamarchuk IS, Slavich GM, Vaillancourt T, Rajji TK. Palamarchuk IS, et al. BMC Neurosci. 2023 Dec 12;24(1):65. doi: 10.1186/s12868-023-00831-2. BMC Neurosci. 2023. PMID: 38087196 Free PMC article. Review.
-
Palamarchuk IS, Vaillancourt T. Palamarchuk IS, et al. Front Behav Neurosci. 2021 Aug 6;15:719674. doi: 10.3389/fnbeh.2021.719674. eCollection 2021. Front Behav Neurosci. 2021. PMID: 34421556 Free PMC article.
References
-
- Adler GK, Smas CM, Fiandaca M, Frim DM, Majzoub JA. Regulated expression of the human corticotropin releasing hormone gene by cyclic AMP. Molecular and Cellular Endocrinology. 1990;70:165–174. - PubMed
-
- Aguilera G, Young WS, Kiss A, Bathia A. Direct regulation of hypothalamic corticotropin-releasing-hormone neurons by angiotensin II. Neuroendocrinology. 1995;61:437–444. - PubMed
-
- Aguilera G. Regulation of Pituitary ACTH Secretion during Chronic Stress. Front Neuroendocrinol. 1994;15:321–350. - PubMed
-
- Aguilera G. Corticotropin Releasing Hormone, Receptor Regulation and the Stress Response. Trends Endocrinol Metab. 1998;9:329–336. - PubMed
-
- Aguilera G, Kiss A, Liu Y, Kamitakahara A. Negative regulation of corticotropin releasing factor expression and limitation of stress response. Stress. 2007;10:153–161. - PubMed
Publication types
MeSH terms
Substances
LinkOut - more resources
Full Text Sources