Models for Golgi traffic: a critical assessment - PubMed
- ️Sat Jan 01 2011
Review
Models for Golgi traffic: a critical assessment
Benjamin S Glick et al. Cold Spring Harb Perspect Biol. 2011.
Abstract
A variety of secretory cargoes move through the Golgi, but the pathways and mechanisms of this traffic are still being debated. Here, we evaluate the strengths and weaknesses of five current models for Golgi traffic: (1) anterograde vesicular transport between stable compartments, (2) cisternal progression/maturation, (3) cisternal progression/maturation with heterotypic tubular transport, (4) rapid partitioning in a mixed Golgi, and (5) stable compartments as cisternal progenitors. Each model is assessed for its ability to explain a set of key observations encompassing multiple cell types. No single model can easily explain all of the observations from diverse organisms. However, we propose that cisternal progression/maturation is the best candidate for a conserved core mechanism of Golgi traffic, and that some cells elaborate this core mechanism by means of heterotypic tubular transport between cisternae.
Figures
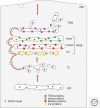
Anterograde vesicular transport between stable compartments. Secretory cargoes travel from the ER to the intermediate compartment (IC) and cis-Golgi in dissociative carriers. Golgi compartments are stable and biochemically distinct. Secretory cargoes move across the stack by means of COPI vesicles that bud from one compartment and fuse with the next, whereas resident Golgi proteins stay in place by being excluded from budding vesicles. This model does not provide a mechanism for transporting secretory cargoes that are too large to fit within COPI vesicles.
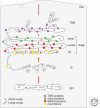
Cisternal progression/maturation. Secretory cargoes exit the ER in dissociative carriers, which coalesce with one another and with COPI vesicles derived from the cis-Golgi to form the intermediate compartment, which coalesces in turn to form a new cis-cisterna. In subsequent rounds of COPI-mediated recycling, the new cisterna matures by receiving medial and then trans-Golgi proteins from older cisternae while exporting cis and then medial-Golgi proteins to younger cisternae. Meanwhile, the cisterna progresses through the stack, carrying forward both small and large secretory cargoes. In the final stage of maturation, the cisterna is a TGN element that breaks down into anterograde and retrograde transport carriers.
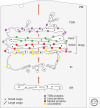
Cisternal progression/maturation with heterotypic tubular transport. This model is identical to the cisternal progression/maturation model, except that cisternae within a given stack are connected by tubular continuities through which small secretory cargoes and resident Golgi proteins can diffuse. Tubular continuities may also exist between heterotypic cisternae in adjacent stacks (not shown).
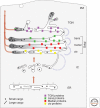
Rapid partitioning in a mixed Golgi. As soon as secretory cargoes enter the Golgi, they equilibrate across the stack via intercisternal continuities. Secretory cargoes partition dynamically between processing domains, which contain resident Golgi proteins, and export domains. These various domains are established by segregation of different lipid species. Secretory cargoes can exit the Golgi at every level of the stack.
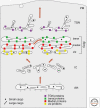
Stable compartments as cisternal progenitors. Secretory cargoes travel from the ER to the intermediate compartment and cis-Golgi in dissociative carriers. Golgi compartments are stable and biochemically distinct, but can segregate into domains by a Rab conversion process. A domain in a cis-cisterna undergoes Rab conversion and acquires medial character, resulting in a transient “homotypic” fusion with a medial cisterna in an adjacent stack. Small and large secretory cargoes can move forward through the resulting connection. Similar domain fusion events in later compartments enable secretory cargoes to traverse the entire stack.
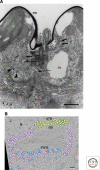
Two views of Golgi stacks in the alga Scherffelia dubia. (A) Thin-section electron micrograph of the apical region of a S. dubia cell. The two Golgi stacks (G) are separated by the nucleus (n). Small arrowheads indicate the transitional ER, which is the site of ER exit. Electron-dense scales can be seen in Golgi cisternae but not in peri-Golgi vesicles. Scale bar, 1.0 µm. (Panel A adapted from Perasso et al. [2000] and reprinted with permission from Springer ©2000.) (B) An electron tomographic slice of a single S. dubia Golgi stack next to a transitional ER site (tER). Superimposed on this slice is a 3D model of the Golgi-associated vesicles, which were interpreted as falling into five classes: two morphologically distinct classes of COPI vesicles (purple and green), COPII vesicles (gold), secretory vesicles (blue), and clathrin-coated vesicles (pink). Scale bar, 100 nm. (Panel B reproduced, with permission, from Staehelin and Kang [2008].)
Similar articles
-
Traffic through the Golgi apparatus.
Pelham HR. Pelham HR. J Cell Biol. 2001 Dec 24;155(7):1099-101. doi: 10.1083/jcb.200110160. Epub 2001 Dec 24. J Cell Biol. 2001. PMID: 11756463 Free PMC article. Review.
-
Live imaging of yeast Golgi cisternal maturation.
Matsuura-Tokita K, Takeuchi M, Ichihara A, Mikuriya K, Nakano A. Matsuura-Tokita K, et al. Nature. 2006 Jun 22;441(7096):1007-10. doi: 10.1038/nature04737. Epub 2006 May 14. Nature. 2006. PMID: 16699523
-
Golgi and related vesicle proteomics: simplify to identify.
Gannon J, Bergeron JJ, Nilsson T. Gannon J, et al. Cold Spring Harb Perspect Biol. 2011 Dec 1;3(12):a005421. doi: 10.1101/cshperspect.a005421. Cold Spring Harb Perspect Biol. 2011. PMID: 21813401 Free PMC article. Review.
-
Small cargoes pass through synthetically glued Golgi stacks.
Dancourt J, Zheng H, Bottanelli F, Allgeyer ES, Bewersdorf J, Graham M, Liu X, Rothman JE, Lavieu G. Dancourt J, et al. FEBS Lett. 2016 Jun;590(12):1675-86. doi: 10.1002/1873-3468.12210. Epub 2016 May 31. FEBS Lett. 2016. PMID: 27174538 Free PMC article.
-
A cisternal maturation mechanism can explain the asymmetry of the Golgi stack.
Glick BS, Elston T, Oster G. Glick BS, et al. FEBS Lett. 1997 Sep 8;414(2):177-81. doi: 10.1016/s0014-5793(97)00984-8. FEBS Lett. 1997. PMID: 9315681 Review.
Cited by
-
Yeong FM. Yeong FM. Bioessays. 2013 May;35(5):462-71. doi: 10.1002/bies.201200144. Epub 2013 Mar 12. Bioessays. 2013. PMID: 23494566 Free PMC article.
-
Nonequilibrium description of de novo biogenesis and transport through Golgi-like cisternae.
Sachdeva H, Barma M, Rao M. Sachdeva H, et al. Sci Rep. 2016 Dec 19;6:38840. doi: 10.1038/srep38840. Sci Rep. 2016. PMID: 27991496 Free PMC article.
-
The Close Relationship between the Golgi Trafficking Machinery and Protein Glycosylation.
Frappaolo A, Karimpour-Ghahnavieh A, Sechi S, Giansanti MG. Frappaolo A, et al. Cells. 2020 Dec 10;9(12):2652. doi: 10.3390/cells9122652. Cells. 2020. PMID: 33321764 Free PMC article. Review.
-
Using glyco-engineering to produce therapeutic proteins.
Dicker M, Strasser R. Dicker M, et al. Expert Opin Biol Ther. 2015;15(10):1501-16. doi: 10.1517/14712598.2015.1069271. Epub 2015 Jul 14. Expert Opin Biol Ther. 2015. PMID: 26175280 Free PMC article. Review.
-
The cellular pathways that maintain the quality control and transport of diverse potassium channels.
Nguyen NH, Brodsky JL. Nguyen NH, et al. Biochim Biophys Acta Gene Regul Mech. 2023 Mar;1866(1):194908. doi: 10.1016/j.bbagrm.2023.194908. Epub 2023 Jan 10. Biochim Biophys Acta Gene Regul Mech. 2023. PMID: 36638864 Free PMC article.
References
-
- Becker B, Bolinger B, Melkonian M 1995. Anterograde transport of algal scales through the Golgi complex is not mediated by vesicles. Trends Cell Biol 5: 305–307 - PubMed
Publication types
MeSH terms
LinkOut - more resources
Full Text Sources