Global proteomic assessment of the classical protein-tyrosine phosphatome and "Redoxome" - PubMed
- ️Sat Jan 01 2011
. 2011 Sep 2;146(5):826-40.
doi: 10.1016/j.cell.2011.07.020.
Minerva Fernandez, Paul Taylor, Carl Virtanen, Jonathan R St-Germain, Lily L Jin, Isaac S Harris, Jun Mori, Tak W Mak, Yotis A Senis, Arne Östman, Michael F Moran, Benjamin G Neel
Affiliations
- PMID: 21884940
- PMCID: PMC3176638
- DOI: 10.1016/j.cell.2011.07.020
Global proteomic assessment of the classical protein-tyrosine phosphatome and "Redoxome"
Robert Karisch et al. Cell. 2011.
Abstract
Protein-tyrosine phosphatases (PTPs), along with protein-tyrosine kinases, play key roles in cellular signaling. All Class I PTPs contain an essential active site cysteinyl residue, which executes a nucleophilic attack on substrate phosphotyrosyl residues. The high reactivity of the catalytic cysteine also predisposes PTPs to oxidation by reactive oxygen species, such as H(2)O(2). Reversible PTP oxidation is emerging as an important cellular regulatory mechanism and might contribute to diseases such as cancer. We exploited these unique features of PTP enzymology to develop proteomic methods, broadly applicable to cell and tissue samples, that enable the comprehensive identification and quantification of expressed classical PTPs (PTPome) and the oxidized subset of the PTPome (oxPTPome). We find that mouse and human cells and tissues, including cancer cells, display distinctive PTPomes and oxPTPomes, revealing additional levels of complexity in the regulation of protein-tyrosine phosphorylation in normal and malignant cells.
Copyright © 2011 Elsevier Inc. All rights reserved.
Figures
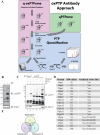
(A) Scheme for each assay. (B) NIH3T3 cells were lysed in presence of DTT, applied to a gel filtration column, treated with PV or left untreated, and then analyzed by immunoblotting with the oxPTP Ab. (C) Native and denatured PV-treated lysates from (B) were immunoprecipitated with oxPTP or Ptpn11 antibodies, as indicated, and analyzed by immunoblotting. (D) PTPs in NIH3T3 cells, as identified by qPTPome. (E) Venn diagram demonstrating reproducibility of the qPTPome assay (n=3). See Figure S1 for additional variations of these assays.
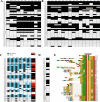
The indicated mouse (A) and human (B) cell lines and tissues were profiled by qPTPome. Classical PTPs identified are shown as black boxes. (C) Comparison of qPTPome results (blue boxes) with published microarray data (black boxes). Correlations for each PTP were evaluated by Spearman r coefficient, as represented by the black-red scale. (D) PTPs in human platelets, as identified by qPTPome. (E) Multiple sequence alignment showing sequence of tryptic fragments containing the catalytic cysteines of each classical PTP. PTPs identified by qPTPome are bolded. Also, see Figure S2.
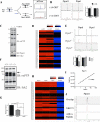
(A) Ptpn11fl/KO cells stably expressing CreER were treated with 4-OHT or left untreated, then assessed by qPTPome. (B) Representative SRM profiles for Ptpn11 and Ptpn1. Quantification of each PTP is displayed next to its elution profile (*** p<0.002, paired Student's t-test; 2 biological replicates, each with 2 technical replicates). (C) Immunoblot showing PTP expression levels. Arrow shows position of Ptpn11. (D) Heat map showing relative changes (compared with untreated cells) in PTP expression (measured by qPTPome) following 4-OHT treatment. The intensity value is the average signal for the SRM profiles for each PTP in untreated cells (E) Ptprd+/+, Ptprd+/− and Ptprd−/− brains were profiled by qPTPome (n=3; 3 technical replicates each). Representative SRM profiles are shown for Ptprd and Ptpn1, with quantification of each displayed next to the profiles. Data represent mean ± SEM (* p<0.05; ** p<0.01; *** p<0.001, ANOVA with Bonferroni post-test). (F) Immunoblot with oxPTP Ab showing PTP expression in Ptprd mutant brains. Arrow shows position of Ptprd. (G) Ptprd mRNA levels (assessed by qPCR; n=3). Data represent mean ± SEM (*** p<0.001, ANOVA with Bonferroni post-test). (H) Relative levels of PTP expression (measured by qPTPome) in Ptprd mutant (compared with Ptprd+/+) brains. (I) Standard curve of MS peak area for Ptpn11 AQUA peptide (n=3). (J) SRM peak of the AQUA peptide mixed with native Ptpn11 peptide from NIH3T3 cells. Also, see Figure S3.
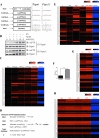
(A) Experimental scheme (left). NIH3T3 cells were stimulated with the indicated concentrations of H2O2 for 5 min and analyzed by q-oxPTPome (n=2; 4 technical replicates each). Representative SRM profiles for Ptpn6 and Ptpn11 (right). (B) Detection of Ptpn6 and Ptpn11 oxidation. Following H2O2 stimulation, cells were processed by q-oxPTPome, immunoprecipitated with Ptpn6 or Ptpn11 antibodies and analyzed by immunoblotting, as indicated. (C) Heat map displaying levels of PTP oxidation following H2O2 stimulation, measured by q-oxPTPome. A black box indicates low levels of PTP oxidation; red boxes denote high levels. The intensity value is the average signal for the SRM profiles for each PTP measured by qPTPome. (D) Scheme showing modifications of qPTPome and q-oxPTPome to assess all potential PTP oxidation states (n=2; each with 3 technical replicates); * indicates a buffer exchange. (E) Fraction of each PTP in each potential oxidation state (i.e. S−, SOH, SO2H, SO3H) following 10 mM H2O2 stimulation. (F) ROS levels in NIH3T3 cells treated with 100 μM BSO (n=6 *** p<0.001, Student's t-test). Data represent mean ± SEM. (G) Levels of PTP oxidation in cells treated with 100 μM BSO or left untreated (n=2; 2 technical replicates each). * indicates PTPs with increased oxidation following BSO treatment. (H) Levels of nitrosylation measured by q-oxPTPome following stimulation with S-nitroso-N-penicillamine (SNAP), S-nitrosoglutathione (GSNO) or S-nitrosocysteine (CSNO). * indicates PTPs that were nitrosylated.
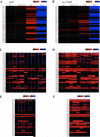
Heat map showing basal PTP oxidation in A431 (A) and Src Y527F-transformed NIH3T3 (B) cells, measured by q-oxPTPome (n=2; 2 technical replicates each). “Negative control” represents cells that were lysed in the presence of DTT, treated with NEM followed by PV, and analyzed by LC-MS/SRM. (C) Basal PTP oxidation in cancer lines determined by q-oxPTPome (n=2; 2 technical replicates each). (D) Same as (C), but with scale altered to show PTPs that were oxidized to lower extents. (E) Basal PTP oxidation in HER2+ breast cancer lines measured by qoxPTPome (n=2; 2 technical replicates each). (F) Same as (E), but with scale altered to show PTPs that were oxidized to lower extents. Also, see Figure S4.
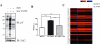
(A) Anti-phosphotyrosine immunoblot and (B) ROS levels of untransformed and Src Y527F cells treated with or without PP2, respectively. Data represent mean ± SEM (n=3; *** p<0.001, ANOVA with Bonferroni post-test). (C) Heat map showing effects of PP2 on levels of PTP oxidation in Src Y527F cells (n=2; 2 technical replicates each). * indicates PTPs with decreased oxidation following PP2 treatment.
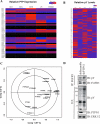
(A) Heat map showing relative PTP expression (based on z-score across all lines) measured by qPTPome in a panel of MM cell lines (n=2, 2 technical replicates each). Black box indicates PTPs that were not identified in any line. (B) Relative phosphotyrosine peptide levels (based on z-score across all MM lines) in the same cells (n=2). (C) Correlation loadings plot displaying results of the PLSR analysis. (D) Lysates from KMS11 cells stably infected with scrambled or PTPN1 shRNAs were immunoprecipated with FGFR3 antibodies and immunoblotted with anti-phosphotyrosine or -FGFR3 antibodies (top). Immunoblots of total cell lystate (TCL) with the indicated antibodies (bottom).
Similar articles
-
Methods to monitor classical protein-tyrosine phosphatase oxidation.
Karisch R, Neel BG. Karisch R, et al. FEBS J. 2013 Jan;280(2):459-75. doi: 10.1111/j.1742-4658.2012.08626.x. Epub 2012 May 30. FEBS J. 2013. PMID: 22577968 Free PMC article. Review.
-
Boivin B, Zhang S, Arbiser JL, Zhang ZY, Tonks NK. Boivin B, et al. Proc Natl Acad Sci U S A. 2008 Jul 22;105(29):9959-64. doi: 10.1073/pnas.0804336105. Epub 2008 Jul 16. Proc Natl Acad Sci U S A. 2008. PMID: 18632564 Free PMC article.
-
Proteomic approaches to studying protein tyrosine phosphatases.
Liang F, Kumar S, Zhang ZY. Liang F, et al. Mol Biosyst. 2007 May;3(5):308-16. doi: 10.1039/b700704n. Epub 2007 Mar 21. Mol Biosyst. 2007. PMID: 17460790 Review.
-
Boivin B, Tonks NK. Boivin B, et al. Methods Enzymol. 2010;474:35-50. doi: 10.1016/S0076-6879(10)74003-9. Epub 2010 Jun 20. Methods Enzymol. 2010. PMID: 20609903
Cited by
-
Xue L, Geahlen RL, Tao WA. Xue L, et al. Mol Cell Proteomics. 2013 Oct;12(10):2969-80. doi: 10.1074/mcp.O113.027722. Epub 2013 Jun 22. Mol Cell Proteomics. 2013. PMID: 23793017 Free PMC article.
-
Functional Analysis of Protein Tyrosine Phosphatases in Thrombosis and Hemostasis.
Rahmouni S, Hego A, Delierneux C, Wéra O, Musumeci L, Tautz L, Oury C. Rahmouni S, et al. Methods Mol Biol. 2016;1447:301-30. doi: 10.1007/978-1-4939-3746-2_17. Methods Mol Biol. 2016. PMID: 27514813 Free PMC article.
-
Circadian redox and metabolic oscillations in mammalian systems.
O'Neill JS, Feeney KA. O'Neill JS, et al. Antioxid Redox Signal. 2014 Jun 20;20(18):2966-81. doi: 10.1089/ars.2013.5582. Epub 2013 Nov 22. Antioxid Redox Signal. 2014. PMID: 24063592 Free PMC article. Review.
-
Impaired Glycoprotein VI-Mediated Signaling and Platelet Functional Responses in CD45 Knockout Mice.
Inamdar VV, Kostyak JC, Badolia R, Dangelmaier CA, Manne BK, Patel A, Kim S, Kunapuli SP. Inamdar VV, et al. Thromb Haemost. 2019 Aug;119(8):1321-1331. doi: 10.1055/s-0039-1692422. Epub 2019 Jun 21. Thromb Haemost. 2019. PMID: 31226719 Free PMC article.
-
Faustino RS, Arrell DK, Folmes CD, Terzic A, Perez-Terzic C. Faustino RS, et al. Croat Med J. 2013 Aug;54(4):319-29. doi: 10.3325//cmj.2013.54.319. Croat Med J. 2013. PMID: 23986272 Free PMC article. Review.
References
-
- Agazie YM, Movilla N, Ischenko I, Hayman MJ. The phosphotyrosine phosphatase SHP2 is a critical mediator of transformation induced by the oncogenic fibroblast growth factor receptor 3. Oncogene. 2003;22:6909–6918. - PubMed
-
- Ahn NG, Shabb JB, Old WM, Resing KA. Achieving in-depth proteomics profiling by mass spectrometry. ACS Chem Biol. 2007;2:39–52. - PubMed
-
- Alonso A, Sasin J, Bottini N, Friedberg I, Friedberg I, Osterman A, Godzik A, Hunter T, Dixon J, Mustelin T. Protein tyrosine phosphatases in the human genome. Cell. 2004;117:699–711. - PubMed
Publication types
MeSH terms
Substances
Grants and funding
LinkOut - more resources
Full Text Sources
Other Literature Sources
Molecular Biology Databases