Antimutator variants of DNA polymerases - PubMed
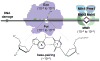
Determinants of DNA replication fidelity. Accurate DNA synthesis is derived from stability differences between correct and incorrect base-pairs (base-pairing), polymerase selectivity (Pol), 3′→5′ exonucleolytic proofreading (Exo), and post-replicative mismatch repair (MMR). Reductions in mutation rate attributed to each mechanism are shown in parentheses. For proofreading, the primer strand partitions to a separate Exo active site, either integral to the polymerase or associated with the holoenzyme. In yeast, the MMR recognition complex is composed of MutS homologues (Msh) 2 and 6 (or a related complex, Msh2 and 3), which recruits an effector complex composed of MutL homologue (Mlh) 1 and post-meiotic segregation (PMS) 1 (both MutL homologues). The background mutation rate may also be influenced by damage to nucleotide pools or template DNA (X). A color version of this figure is available online.
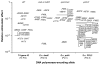
Antimutator effects of DNA polymerase variants. The relative mutation frequency or rate conferred by T4 Pol, E. coli Pol III and Pol I, and yeast Pol δ antimutator variants are shown. Each variant is indicated by the single amino-acid substitution that confers antimutator activity. The T4 Pol antimutator effects are based on reversion frequency of rII UV199oc relative to wild-type T4 Pol (Reha-Krantz, 1988; Reha-Krantz and Nonay, 1993; Reha-Krantz and Nonay, 1994; Reha-Krantz and Wong, 1996). The value for the S411T single mutant is inferred from the reversion frequency of the S411T, L412M double mutant (Reha-Krantz and Nonay, 1993). Pol III antimutator effects are based on the average degree of mutL and/or mutD5 mutator suppression, as measured by the frequency of Rifampicin-resistance, Nalidixic acid-resistance, GalK2 (ochre) reversion and/or lacZ118 (ochre) reversion (Fijalkowska et al., 1993; Fijalkowska and Schaaper, 1993; Fijalkowska and Schaaper, 1995; Fijalkowska and Schaaper, 1996). The average background reversion frequency for WT cells is reported to be 3 × 10−4 relative to mutD5 (Schaaper, 1988). All Pol I antimutators were engineered into an E. coli Pol I variant lacking proofreading capability (D424A), with the exception of the Taq Pol I antimutator F667L (Suzuki et al., 2000). The relative antimutator activities of Pol I variants marked with an asterisk are based on in vitro gap-filling mutation rate determinations (Minnick et al., 1999). All other Pol I values are based on reversion frequencies of a premature nonsense codon in a plasmid-borne β-lactamase gene (Suzuki et al., 2000; Shinkai and Loeb, 2001; Shinkai et al., 2001; Loh et al., 2007). The effect of Pol δ antimutators are based on suppression of the proofreading-defective pol3-01 mutator allele, as measured by the rate of FOA-resistance mutations (Herr et al., 2011).
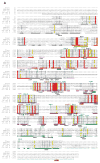
B-family polymerase antimutators. (A) Aligned sequences of five B-family DNA polymerases are shown: bacteriophage T4 (T4 Pol), bacteriophage RB69 (RB69 pol), Herpes Simplex Virus 1 (HSV1 pol), Thermococcus gorgonarius (T.g. pol B), and Saccharomyces cerevisiae (S.c. pol d). Secondary structural elements of yeast Pol δ (Swan et al., 2009) are indicated below the alignment and color coded to depict their locations in the amino (gray), exo (red), palm (purple), fingers (blue) and thumb (green) domains of the protein: rectangles, α-helices; arrows, β-strands; solid lines, loops; dashed lines, structure not solved. Highlighted amino-acid residues in the alignment correspond to the following: red, absolutely conserved; yellow, identical in majority of sequences; gray, similar in majority of sequences. Conserved polymerase and exonuclease motifs are indicated by colored frames: blue, Exo motifs; green, Pol motifs (Wang et al., 1989); brown, motifs A, B, C (Delarue et al., 1990). Amino-acid substitutions of interest are placed above or below the alignment at the relevant position, highlighted according to the following scheme: aqua, T4 Pol antimutators (Reha-Krantz, 1995a; Reha-Krantz and Wong, 1996; Reha-Krantz, 2010); magenta, HSV1 Pol antimutators (Hall et al., 1984; Gibbs et al., 1988; Hwang et al., 2004; Tian et al., 2009); yellow, yeast pol3-01; no highlight, antimutators isolated as suppressors of the pol3-01 mutator phenotype; green, antimutators isolated as suppressors of the pol3-D407A mutator phenotype; orange, antimutators isolated as suppressors of the pol3-F406A mutator phenotype (three substitutions in the same mutant); blue, antimutator isolated as a suppressor of the pol3-D463A mutator phenotype; gray, previously reported antimutators in Pol δ (pol3-t (D643N), G447S, Y708A, and V758M) (Tran et al., 1999a; Hadjimarcou et al., 2001; Pavlov et al., 2004; Li et al., 2005). The antimutators affecting pol3-01, pol3-D407A, pol3-F406A, and pol3-D463A are from Herr et al. (Herr et al., 2011). (B-I) Locations of antimutator amino-acid substitutions in T4 Pol [B, D, F, and H; mapped onto RB69Pol (Franklin et al., 2001), Protein Data Bank accession code 1IG9] and Pol δ [C, E, G, and I; mapped onto Pol δ (Swan et al., 2009), PDB accession code 3IAY]. The proteins are shown as schematic diagrams of the α-carbon backbone with α-carbons or amino acids of interest depicted as space-filling spheres. Wild type amino-acid residues corresponding to antimutator mutations are shown as teal spheres and labeled to indicate the antimutator substitutions. Structural domains are color coded as in the alignment in panel A. The incoming dNTPs are denoted by green CPK sticks and the template nucleotides by orange CPK sticks. Active-site carboxylate side chains are gray CPK sticks coming out of the purple (palm) or red (exo) ribbons. Metal ions are small black spheres. Important non-mutated residues proximal to the antimutator substitutions are also shown as space-filling spheres and color coded according to domain. (B and C) Distribution of antimutator substitutions. The α-carbons of wild type residues corresponding to antimutators are shown as teal spheres. (D and E) Antimutator substitutions near the polymerase active site. The fingers and thumb domains have been removed for clarity. T4 Pol S345 and Pol δ residues V546, G555, I558, and Q563 from the amino domain are in three closely associated α-helices that bind the template and buttress the fingers domain. The antimutator substitution (V758M) that conferred PAA-resistance to a Pol δ-L612M strain is shown in yellow. (F and G) Antimutator substitutions in the exo domain. All T4 Pol antimutator variants occurred in the context of a functional exonuclease, unlike Pol δ antimutator polymerases, which carried D321A and D323A substitutions that inactivate the exonuclease. The dotted red line in (G) corresponds to a missing loop in the Pol δ structure (amino acids 491–496). (H and I) Antimutator substitutions in the DNA binding track. The primer (gold) and template (brown) are held by a series of interactions along the DNA minor groove. Minor-groove ‘sensing’ residues in the KKRYA motif of the palm domain (T4 Pol K702, K703, R704; Pol δ K813, K814 and R815) are shown as space-filling spheres labeled K and R. Unpaired 5′ nucleotides of the template along with the amino, exo, and fingers domains have been removed for clarity. A color version of this figure is available online.
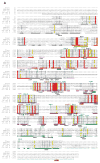
B-family polymerase antimutators. (A) Aligned sequences of five B-family DNA polymerases are shown: bacteriophage T4 (T4 Pol), bacteriophage RB69 (RB69 pol), Herpes Simplex Virus 1 (HSV1 pol), Thermococcus gorgonarius (T.g. pol B), and Saccharomyces cerevisiae (S.c. pol d). Secondary structural elements of yeast Pol δ (Swan et al., 2009) are indicated below the alignment and color coded to depict their locations in the amino (gray), exo (red), palm (purple), fingers (blue) and thumb (green) domains of the protein: rectangles, α-helices; arrows, β-strands; solid lines, loops; dashed lines, structure not solved. Highlighted amino-acid residues in the alignment correspond to the following: red, absolutely conserved; yellow, identical in majority of sequences; gray, similar in majority of sequences. Conserved polymerase and exonuclease motifs are indicated by colored frames: blue, Exo motifs; green, Pol motifs (Wang et al., 1989); brown, motifs A, B, C (Delarue et al., 1990). Amino-acid substitutions of interest are placed above or below the alignment at the relevant position, highlighted according to the following scheme: aqua, T4 Pol antimutators (Reha-Krantz, 1995a; Reha-Krantz and Wong, 1996; Reha-Krantz, 2010); magenta, HSV1 Pol antimutators (Hall et al., 1984; Gibbs et al., 1988; Hwang et al., 2004; Tian et al., 2009); yellow, yeast pol3-01; no highlight, antimutators isolated as suppressors of the pol3-01 mutator phenotype; green, antimutators isolated as suppressors of the pol3-D407A mutator phenotype; orange, antimutators isolated as suppressors of the pol3-F406A mutator phenotype (three substitutions in the same mutant); blue, antimutator isolated as a suppressor of the pol3-D463A mutator phenotype; gray, previously reported antimutators in Pol δ (pol3-t (D643N), G447S, Y708A, and V758M) (Tran et al., 1999a; Hadjimarcou et al., 2001; Pavlov et al., 2004; Li et al., 2005). The antimutators affecting pol3-01, pol3-D407A, pol3-F406A, and pol3-D463A are from Herr et al. (Herr et al., 2011). (B-I) Locations of antimutator amino-acid substitutions in T4 Pol [B, D, F, and H; mapped onto RB69Pol (Franklin et al., 2001), Protein Data Bank accession code 1IG9] and Pol δ [C, E, G, and I; mapped onto Pol δ (Swan et al., 2009), PDB accession code 3IAY]. The proteins are shown as schematic diagrams of the α-carbon backbone with α-carbons or amino acids of interest depicted as space-filling spheres. Wild type amino-acid residues corresponding to antimutator mutations are shown as teal spheres and labeled to indicate the antimutator substitutions. Structural domains are color coded as in the alignment in panel A. The incoming dNTPs are denoted by green CPK sticks and the template nucleotides by orange CPK sticks. Active-site carboxylate side chains are gray CPK sticks coming out of the purple (palm) or red (exo) ribbons. Metal ions are small black spheres. Important non-mutated residues proximal to the antimutator substitutions are also shown as space-filling spheres and color coded according to domain. (B and C) Distribution of antimutator substitutions. The α-carbons of wild type residues corresponding to antimutators are shown as teal spheres. (D and E) Antimutator substitutions near the polymerase active site. The fingers and thumb domains have been removed for clarity. T4 Pol S345 and Pol δ residues V546, G555, I558, and Q563 from the amino domain are in three closely associated α-helices that bind the template and buttress the fingers domain. The antimutator substitution (V758M) that conferred PAA-resistance to a Pol δ-L612M strain is shown in yellow. (F and G) Antimutator substitutions in the exo domain. All T4 Pol antimutator variants occurred in the context of a functional exonuclease, unlike Pol δ antimutator polymerases, which carried D321A and D323A substitutions that inactivate the exonuclease. The dotted red line in (G) corresponds to a missing loop in the Pol δ structure (amino acids 491–496). (H and I) Antimutator substitutions in the DNA binding track. The primer (gold) and template (brown) are held by a series of interactions along the DNA minor groove. Minor-groove ‘sensing’ residues in the KKRYA motif of the palm domain (T4 Pol K702, K703, R704; Pol δ K813, K814 and R815) are shown as space-filling spheres labeled K and R. Unpaired 5′ nucleotides of the template along with the amino, exo, and fingers domains have been removed for clarity. A color version of this figure is available online.
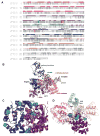
Pol III antimutators. (A) Alignment of Pol III peptide sequences from Thermus aquaticus (Taq Pol III) and Escherichia coli (E.c. Pol III). Secondary structural elements based on the Taq Pol III structure (Wing et al., 2008) (PDB accession code 3E0D) are indicated below the alignment and color coded to depict their location (PHP, magenta; palm, purple; fingers, blue; thumb, green; β-binding, orange; carboxy-terminus, charcoal): rectangles, α-helices; arrows, β-strands; solid lines, loops. Amino acids shaded in gray are conserved between Taq and E. coli. Aspartates that coordinate metal ions for catalysis are shaded in red. Residues that stack with ribose of incoming dNTP are shaded in cyan. E. coli dnaE amino-acid changes and allele numbers are depicted below the alignment. (B) Distribution of antimutator substitutions. The α-carbons of antimutator positions are shown as teal spheres. (C) Antimutators near the Pol III active site. The RGS motif (362–364) and H760 cradle the incoming dNTP, while R390 and R710 interact with the triphosphate. (D) Antimutators in the Pol III Thumb. A498T and P464L affect the positioning of an α-helix that includes three basic residues that interact with the primer•template backbone (K439, R443, R447). A color version of this figure is available online.
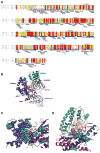
Pol I antimutators. (A) Alignment of Pol I peptide sequences from Bacillus stearothermophilus (Bst Pol I), Thermus aquaticus (Taq Pol I), and Escherichia coli (E.c. Pol I). Secondary structural elements based on the Taq Pol I structure (Li et al., 1998) (PDB accession code 3KTQ) are indicated below the alignment and color coded to depict their locations in the amino (the proofreading exonuclease in E. coli Pol I; gray); palm (purple), thumb (green) and fingers (blue) domains of the protein: rectangles, α-helices; arrows, β-strands; solid lines, loops. Highlighted amino acid residues in the alignment correspond to the following: red, absolutely conserved; yellow, identical in majority of sequences; gray, similar in majority of sequences. Conserved Pol I motifs are indicated by green frames. Amino-acid substitutions of interest are placed below the alignment at the relevant position, color coded according to the following scheme: teal, single amino acid antimutator substitutions in E. coli Pol I (Minnick et al., 1999; Loh et al., 2007); black, substitutions found in complex mutant alleles from Loh et. al (Loh et al., 2007); white lettering with teal shaded box, Taq Pol I antimutator, (Suzuki et al., 2000). (B) Distribution of antimutator substitutions. The α-carbons of antimutator positions are shown as teal spheres. (C) Pol I active site antimutator substitutions. (D) Pol I antimutators that may influence DNA binding. Purple spheres next to L676Q correspond to adjacent amino acids from Motif 2 that bind the -3 base pair of the primer•template (PNL676QN). A color version of this figure is available online.
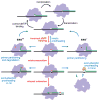
Mechanisms of DNA polymerase fidelity and antimutagenesis. A reiterative cycle of correct dNTP binding, nucleotide incorporation, and translocation comprises accurate DNA replication (top, black arrows). Antimutator mechanisms (blue arrows) enhance existing processes that oppose incorrect dNTP binding and misincorporation (red arrows) in DNA polymerases with functional (Exo+) or defective intrinsic proofreading (Exo−). Antimutator substitutions may increase the effectiveness of kinetic proofreading, by reducing incorrect dNTP binding stability or by enhancing partitioning of correctly paired primer strands to the exonuclease active site in the presence of bound, incorrect dNTPs. In proofreading-proficient polymerases, partitioning to the exonuclease domain may lead to degradation of correct primer ends, as exemplified by T4 Pol optA1 sensitivity, as well as improved editing of misincorporations. In the absence of intrinsic proofreading, antimutators may enhance polymerase dissociation from the primer•template DNA, leading to editing by other cellular 3′→5′ exonucleases (proofreading in trans). A color version of this figure is available online.