Slit/Robo-mediated axon guidance in Tribolium and Drosophila: divergent genetic programs build insect nervous systems - PubMed
- ️Sun Jan 01 2012
Slit/Robo-mediated axon guidance in Tribolium and Drosophila: divergent genetic programs build insect nervous systems
Timothy A Evans et al. Dev Biol. 2012.
Abstract
As the complexity of animal nervous systems has increased during evolution, developmental control of neuronal connectivity has become increasingly refined. How has functional diversification within related axon guidance molecules contributed to the evolution of nervous systems? To address this question, we explore the evolution of functional diversity within the Roundabout (Robo) family of axon guidance receptors. In Drosophila, Robo and Robo2 promote midline repulsion, while Robo2 and Robo3 specify the position of longitudinal axon pathways. The Robo family has expanded by gene duplication in insects; robo2 and robo3 exist as distinct genes only within dipterans, while other insects, like the flour beetle Tribolium castaneum, retain an ancestral robo2/3 gene. Both Robos from Tribolium can mediate midline repulsion in Drosophila, but unlike the fly Robos cannot be down-regulated by Commissureless. The overall architecture and arrangement of longitudinal pathways are remarkably conserved in Tribolium, despite it having only two Robos. Loss of TcSlit causes midline collapse of axons in the beetle, a phenotype recapitulated by simultaneous knockdown of both Robos. Single gene knockdowns reveal that beetle Robos have specialized axon guidance functions: TcRobo is dedicated to midline repulsion, while TcRobo2/3 also regulates longitudinal pathway formation. TcRobo2/3 knockdown reproduces aspects of both Drosophila robo2 and robo3 mutants, suggesting that TcRobo2/3 has two functions that in Drosophila are divided between Robo2 and Robo3. The ability of Tribolium to organize longitudinal axons into three discrete medial-lateral zones with only two Robo receptors demonstrates that beetle and fly achieve equivalent developmental outcomes using divergent genetic programs.
Copyright © 2012 Elsevier Inc. All rights reserved.
Figures
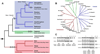
A. Schematic phylogeny showing the evolutionary relationship between selected animals, and the complement of Robo receptors present in each animal’s genome. Predicted gene duplication events are represented by open circles. Filled black circle represents the appearance of the ancestral Robo receptor. The Robo family has expanded via gene duplication in insects (blue), crustaceans (green), and vertebrates (red). Daphnia Robos are given numerical designation based on the genomic contig in which they are located. Branch lengths are not to scale. B. Phylogenetic analysis of Robo family members using extracellular (Ig+Fn) protein sequences. Insect (blue), crustacean (green), and vertebrate (red) receptors cluster together, reflecting their independent derivation from a single common ancestral receptor. Note also that Robo2 and Robo3 orthologs in fly (Dm) and mosquito (Ag) are more similar to each other than to Robo2/3 from beetle (Tc) and louse (Ph). A single Robo receptor (SAX-3) has been retained in nematodes. Abbreviations: Dm, Drosophila melanogaster; Ag, Anopheles gambiae; Tc, Tribolium castaneum; Ph, Pediculus humanus; Dp, Daphnia pulex; Ce, Caenorhabditis elegans; Hs, Homo sapiens; Mm, Mus musculus; Dr, Danio rerio. C. Schematic comparison of Drosophila and Tribolium Robo receptors showing percent sequence identity between individual protein domains, and highlighting the conserved cytoplasmic (CC) motifs. Lengths of cytodomains are roughly to scale. TcRobo retains all four CC motifs despite its much smaller cytodomain. Note that Drosophila Robo2 and Robo3 are more similar to each other than to Tribolium Robo2/3.
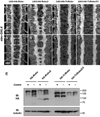
A–D. Stage 16–17 Drosophila embryos carrying elav-GAL4 and the indicated UAS-Robo transgenes. In this and all subsequent figures, all UAS-Robo transgenes have identical 5’UTR, signal sequence and 3×HA N-terminal tags, and are inserted into the same genomic location (at cytological position 86FB). Anti-HA staining shows that Robo receptors from fly and beetle localize to axons when expressed pan-neurally in the Drosophila embryonic CNS (A–D). Tribolium Robos are much more effective at preventing axons from crossing the midline than Drosophila Robos, producing a commissureless phenotype as revealed by anti-HRP staining (A′–D′). E. Western blot of total lysates from 0–24 hour embryos carrying elav-GAL4 and the indicated UAS-Robo transgenes, with or without UAS-Comm. Lysates were subjected to SDS-PAGE and immunoblotted with anti-HA to detect the levels of HA-tagged receptors, and anti-tubulin as a loading control. Levels of HA-tagged Drosophila Robo and Robo2 are strongly reduced in the presence of exogenous Comm, while the levels of HA-tagged TcRobo and TcRobo2/3 were similar in the presence or absence of exogenous Comm.
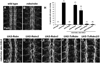
A–G. Stage 16–17 Drosophila embryos stained with mAb 1D4 (anti-FasII). A. Wild type embryo. FasII-positive axon pathways do not cross the midline. B. In robo mutant embryos, axons from the medial FasII pathways cross the midline in every segment. C–G. robo mutant embryos carrying elav-GAL4 and indicated UAS-Robo transgenes. C. Pan-neural expression of Drosophila Robo fully rescues the robo loss of function phenotype: FasII-positive axons no longer cross the midline. D,E. Expression of Drosophila Robo2 (D) or Robo3 (E) does not prevent ectopic midline crossing in a robo mutant background. F,G. Expression of Tribolium Robo (D) or Tribolium Robo2/3 (E) is sufficient to prevent ectopic midline crossing in the absence of endogenous robo. H. Quantification of ectopic midline crossing (average percent segments with ectopic FasII crossing per embryo) in the genetic backgrounds shown in A–G. Error bars represent s.e.m. Number of embryos scored for each genotype is indicated in parentheses.
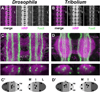
A–B. Dissected ventral nerve cords from a stage 16–17 Drosophila embryo (A) and 4–5 day old Tribolium embryo (B) stained with antibodies against HRP (magenta; detects all axons in the CNS) and the longitudinal pathway marker FasciclinII (FasII; green). Both insects exhibit a ladder-like arrangement of axon pathways in the CNS consisting of bilateral longitudinal connectives and two commissures per segment. Anterior and posterior commissures in a single segment are indicated by white and black arrowheads, respectively. Individual FasII-positive axon pathways are visible in both species, and they do not cross the midline. Peripheral nerves are labeled by both anti-HRP and anti-FasII in each species as they exit the CNS (arrows). C–D. Single abdominal segments of Drosophila (C) and Tribolium (D) embryos stained as in A–B. Maximum confocal projections through the entire neuropile are shown above; optical cross sections taken at the level of the white hash mark are shown below. (C′,D′). Schematic representation of cross sections shown in C,D denoting medial (M, light gray), intermediate (I, medium gray) and lateral (L, dark gray) regions of the neuropile and showing relative positions of individual longitudinal pathways (black dots). C–C′. FasII-positive pathways form in three distinct zones within the Drosophila neuropile. At least eight distinct FasII pathways can be seen in cross section: the dorsal medial (DM) and ventral medial (VM) pathways form within the medial (M) zone; three central intermediate (CI) and one dorsal intermediate (DI) pathway form within the intermediate (I) zone; the dorsal lateral (DL) and ventral lateral (VL) pathways form within the lateral (L) zone. Pathway nomenclature is after Landgraf et al 2003. D–D′. Similar arrangement of axon pathways in the Tribolium embryonic CNS. Distinct FasII-positive pathways are visible at medial, intermediate, and lateral positions. The same number of individual pathways can be seen in cross section at equivalent dorsoventral and mediolateral locations to those in Drosophila. In this and all subsequent figures, Tribolium embryos are shown scaled to 75% original size for easier comparison with the smaller Drosophila embryos.
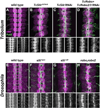
A–D. 4–5 day old Tribolium embryos stained with anti-HRP (magenta) and anti-TcFasII (green). Small panels below show individual HRP (left) and FasII (right) channels. A. Wild type Tribolium embryo. The ladder-like axon scaffold is formed by segmentally repeated pairs of commissures and bilateral longitudinal connectives. Distinct FasII-positive pathways are visible, and they do not cross the midline. B. In TcSlitG07615 embryos, commissures are fused and FasII-positive axons linger at the midline, similar to the phenotype seen in Drosophila hypomorphic slit mutants (F). Some longitudinal pathways still form relatively normally in these embryos. C. Embryos from mothers injected with dsRNA targeting TcSlit display a strong midline collapse phenotype. The width of the axon scaffold is reduced and longitudinal pathways do not form properly, as many FasII-positive axons enter the midline and fail to leave. D. Embryos in which TcRobo and TcRobo2/3 are targeted in combination phenocopy the TcSlit knockdown phenotype. E–H. Stage 16–17 Drosophila embryos stained with anti-HRP (magenta) and mAb 1D4 (anti-FasII; green). E. In wild type Drosophila embryos, the longitudinal connectives are well separated and connected by two commissures per segment. Three distinct FasII-positive pathways can be seen on either side of the midline; they do not cross the midline. F. In hypomorphic slit mutants, the connectives are closer together, commissures thicken and begin to fuse, and FasII-positive axons cross the midline in every segment. G. In slit null mutants, all axons collapse at the midline, reflecting a complete absence of midline repulsion. H. Double mutant robo,robo2 embryos display a slit-like midline collapse phenotype.
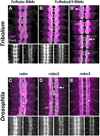
Tribolium (A,B) and Drosophila (C–E) embryos prepared as in Figure 5. A. TcRobo knockdown embryo. Commissures are thickened and FasII axons from medial pathways ectopically cross the midline (arrow). Intermediate and lateral longitudinal pathways are formed relatively normally. B. TcRobo2/3 knockdown embryo. The axon scaffold has an overall normal appearance, and no FasII-positive axons cross the midline. Longitudinal pathways are disorganized and often fail to form at their normally stereotyped positions. B′. Partial confocal projections from four different TcRobo2/3 knockdown embryos displaying longitudinal pathway defects: missing lateral pathways (empty arrowhead); FasII-positive axons wandering between longitudinal pathways (arrowhead with asterisk); intermediate and lateral pathways compressed into medial zone (arrow). In a minority of segments, intermediate and lateral pathways form relatively normally (solid arrowhead). Projections were made from 8–15 0.2 µm confocal sections and include all dorsal pathways in their entirety while excluding the majority of ventral pathways. C. robo mutant embryo. As in TcRobo knockdown embryos (A), thickened commissures and ectopic midline crossing of FasII axons can be seen (arrow). D. robo2 mutant embryo. Although the axon scaffold appears relatively normal, medial FasII axons can occasionally be seen crossing the midline (arrow). In addition, lateral FasII pathways are often absent (empty arrowhead) or fuse with intermediate pathways (arrowhead with asterisk). E. robo3 mutant embryo. The axon scaffold appears normal, and no ectopic crossing is detectable. Intermediate FasII pathways shift towards the midline and fuse with medial pathways (arrow).
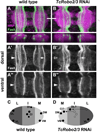
A,B. High-magnification views of single abdominal segments from wild type (A) and TcRobo2/3 knockdown (B) embryos. Maximum confocal projections through the entire neuropile are shown above; optical cross-sections taken at the level of the white hash mark are shown below. A′,B′. Partial confocal projections showing only the dorsal-most FasII pathways. A″,B″. Partial confocal projections showing only the ventral-most FasII pathways. In wild type embryos, a major FasII pathway forms in the dorsal lateral region of the neuropile (solid arrowhead in A′). In TcRobo2/3 knockdown embryos, this pathway is missing in the majority of segments (empty arrowhead in B′). The ventral lateral FasII pathway appears unaffected by the loss of TcRobo2/3 (solid arrowhead in A″, B″). In addition, the 3–4 FasII pathways that normally form in the intermediate region (arrow in A, A′) are shifted medially in TcRobo2/3 knockdown embryos (arrow in B, B′). C,D. Schematic comparison of longitudinal pathways in wild type (C) and TcRobo2/3 knockdown (D) embryos. Loss of TcRobo2/3 results in the absence (dashed circles) or medial shifting (dashed arrows) of both lateral and intermediate pathways. In the absence of more restricted molecular markers, the identity of ectopic pathways forming within the medial zone cannot be determined unless axons can be detected shifting from more lateral pathways. Medial (DM and VM) and ventral lateral (VL) pathways appear unaffected by loss of TcRobo2/3. See Figure 4 legend for longitudinal pathway nomenclature.
Similar articles
-
Evans TA. Evans TA. Evodevo. 2017 Jun 1;8:10. doi: 10.1186/s13227-017-0073-y. eCollection 2017. Evodevo. 2017. PMID: 28588759 Free PMC article.
-
Regulation of axon guidance by slit and netrin signaling in the Drosophila ventral nerve cord.
Bhat KM, Gaziova I, Krishnan S. Bhat KM, et al. Genetics. 2007 Aug;176(4):2235-46. doi: 10.1534/genetics.107.075085. Epub 2007 Jun 11. Genetics. 2007. PMID: 17565966 Free PMC article.
-
Spitzweck B, Brankatschk M, Dickson BJ. Spitzweck B, et al. Cell. 2010 Feb 5;140(3):409-20. doi: 10.1016/j.cell.2010.01.002. Cell. 2010. PMID: 20144763
-
Blockus H, Chédotal A. Blockus H, et al. Development. 2016 Sep 1;143(17):3037-44. doi: 10.1242/dev.132829. Development. 2016. PMID: 27578174 Review.
-
Axon guidance: Robos make the rules.
Guthrie S. Guthrie S. Curr Biol. 2001 Apr 17;11(8):R300-3. doi: 10.1016/s0960-9822(01)00169-5. Curr Biol. 2001. PMID: 11369218 Review.
Cited by
-
Molecular mechanisms regulating axon responsiveness at the midline.
Gorla M, Bashaw GJ. Gorla M, et al. Dev Biol. 2020 Oct 1;466(1-2):12-21. doi: 10.1016/j.ydbio.2020.08.006. Epub 2020 Aug 17. Dev Biol. 2020. PMID: 32818516 Free PMC article.
-
Evans TA. Evans TA. Evodevo. 2017 Jun 1;8:10. doi: 10.1186/s13227-017-0073-y. eCollection 2017. Evodevo. 2017. PMID: 28588759 Free PMC article.
-
Molecular characterization of the netrin-1 UNC-5 receptor in Lucilia sericata larvae.
Karamzadeh T, Alipour H, Shahriari-Namadi M, Raz A, Azizi K, Bagheri M, Moemenbellah-Fard MD. Karamzadeh T, et al. AIMS Genet. 2019 Aug 8;6(3):46-54. doi: 10.3934/genet.2019.3.46. eCollection 2019. AIMS Genet. 2019. PMID: 31663032 Free PMC article.
-
Evolution and development of interhemispheric connections in the vertebrate forebrain.
Suárez R, Gobius I, Richards LJ. Suárez R, et al. Front Hum Neurosci. 2014 Jul 14;8:497. doi: 10.3389/fnhum.2014.00497. eCollection 2014. Front Hum Neurosci. 2014. PMID: 25071525 Free PMC article. Review.
-
Brown HE, Reichert MC, Evans TA. Brown HE, et al. G3 (Bethesda). 2015 Sep 10;5(11):2429-39. doi: 10.1534/g3.115.022327. G3 (Bethesda). 2015. PMID: 26362767 Free PMC article.
References
-
- Bastiani MJ, Harrelson AL, Snow PM, Goodman CS. Expression of fasciclin I and II glycoproteins on subsets of axon pathways during neuronal development in the grasshopper. Cell. 1987;48:745–755. - PubMed
-
- Brand AH, Perrimon N. Targeted gene expression as a means of altering cell fates and generating dominant phenotypes. Development. 1993;118:401–415. - PubMed
-
- Bucher G, Scholten J, Klingler M. Parental RNAi in Tribolium (Coleoptera) Curr Biol. 2002;12:R85–R86. - PubMed
-
- Chen Z, Gore BB, Long H, Ma L, Tessier-Lavigne M. Alternative splicing of the Robo3 axon guidance receptor governs the midline switch from attraction to repulsion. Neuron. 2008;58:325–332. - PubMed
-
- Clark AG, Eisen MB, Smith DR, Bergman CM, Oliver B, Markow TA, Kaufman TC, Kellis M, Gelbart W, Iyer VN, Pollard DA, Sackton TB, Larracuente AM, Singh ND, Abad JP, Abt DN, Adryan B, Aguade M, Akashi H, Anderson WW, Aquadro CF, Ardell DH, Arguello R, Artieri CG, Barbash DA, Barker D, Barsanti P, Batterham P, Batzoglou S, Begun D, Bhutkar A, Blanco E, Bosak SA, Bradley RK, Brand AD, Brent MR, Brooks AN, Brown RH, Butlin RK, Caggese C, Calvi BR, Bernardo de Carvalho A, Caspi A, Castrezana S, Celniker SE, Chang JL, Chapple C, Chatterji S, Chinwalla A, Civetta A, Clifton SW, Comeron JM, Costello JC, Coyne JA, Daub J, David RG, Delcher AL, Delehaunty K, Do CB, Ebling H, Edwards K, Eickbush T, Evans JD, Filipski A, Findeiss S, Freyhult E, Fulton L, Fulton R, Garcia AC, Gardiner A, Garfield DA, Garvin BE, Gibson G, Gilbert D, Gnerre S, Godfrey J, Good R, Gotea V, Gravely B, Greenberg AJ, Griffiths-Jones S, Gross S, Guigo R, Gustafson EA, Haerty W, Hahn MW, Halligan DL, Halpern AL, Halter GM, Han MV, Heger A, Hillier L, Hinrichs AS, Holmes I, Hoskins RA, Hubisz MJ, Hultmark D, Huntley MA, Jaffe DB, Jagadeeshan S, Jeck WR, Johnson J, Jones CD, Jordan WC, Karpen GH, Kataoka E, Keightley PD, Kheradpour P, Kirkness EF, Koerich LB, Kristiansen K, Kudrna D, Kulathinal RJ, Kumar S, Kwok R, Lander E, Langley CH, Lapoint R, Lazzaro BP, Lee SJ, Levesque L, Li R, Lin CF, Lin MF, Lindblad-Toh K, Llopart A, Long M, Low L, Lozovsky E, Lu J, Luo M, Machado CA, Makalowski W, Marzo M, Matsuda M, Matzkin L, McAllister B, McBride CS, McKernan B, McKernan K, Mendez-Lago M, Minx P, Mollenhauer MU, Montooth K, Mount SM, Mu X, Myers E, Negre B, Newfeld S, Nielsen R, Noor MA, O'Grady P, Pachter L, Papaceit M, Parisi MJ, Parisi M, Parts L, Pedersen JS, Pesole G, Phillippy AM, Ponting CP, Pop M, Porcelli D, Powell JR, Prohaska S, Pruitt K, Puig M, Quesneville H, Ram KR, Rand D, Rasmussen MD, Reed LK, Reenan R, Reily A, Remington KA, Rieger TT, Ritchie MG, Robin C, Rogers YH, Rohde C, Rozas J, Rubenfield MJ, Ruiz A, Russo S, Salzberg SL, Sanchez-Gracia A, Saranga DJ, Sato H, Schaeffer SW, Schatz MC, Schlenke T, Schwartz R, Segarra C, Singh RS, Sirot L, Sirota M, Sisneros NB, Smith CD, Smith TF, Spieth J, Stage DE, Stark A, Stephan W, Strausberg RL, Strempel S, Sturgill D, Sutton G, Sutton GG, Tao W, Teichmann S, Tobari YN, Tomimura Y, Tsolas JM, Valente VL, Venter E, Venter JC, Vicario S, Vieira FG, Vilella AJ, Villasante A, Walenz B, Wang J, Wasserman M, Watts T, Wilson D, Wilson RK, Wing RA, Wolfner MF, Wong A, Wong GK, Wu CI, Wu G, Yamamoto D, Yang HP, Yang SP, Yorke JA, Yoshida K, Zdobnov E, Zhang P, Zhang Y, Zimin AV, Baldwin J, Abdouelleil A, Abdulkadir J, Abebe A, Abera B, Abreu J, Acer SC, Aftuck L, Alexander A, An P, Anderson E, Anderson S, Arachi H, Azer M, Bachantsang P, Barry A, Bayul T, Berlin A, Bessette D, Bloom T, Blye J, Boguslavskiy L, Bonnet C, Boukhgalter B, Bourzgui I, Brown A, Cahill P, Channer S, Cheshatsang Y, Chuda L, Citroen M, Collymore A, Cooke P, Costello M, D'Aco K, Daza R, De Haan G, DeGray S, DeMaso C, Dhargay N, Dooley K, Dooley E, Doricent M, Dorje P, Dorjee K, Dupes A, Elong R, Falk J, Farina A, Faro S, Ferguson D, Fisher S, Foley CD, Franke A, Friedrich D, Gadbois L, Gearin G, Gearin CR, Giannoukos G, Goode T, Graham J, Grandbois E, Grewal S, Gyaltsen K, Hafez N, Hagos B, Hall J, Henson C, Hollinger A, Honan T, Huard MD, Hughes L, Hurhula B, Husby ME, Kamat A, Kanga B, Kashin S, Khazanovich D, Kisner P, Lance K, Lara M, Lee W, Lennon N, Letendre F, LeVine R, Lipovsky A, Liu X, Liu J, Liu S, Lokyitsang T, Lokyitsang Y, Lubonja R, Lui A, MacDonald P, Magnisalis V, Maru K, Matthews C, McCusker W, McDonough S, Mehta T, Meldrim J, Meneus L, Mihai O, Mihalev A, Mihova T, Mittelman R, Mlenga V, Montmayeur A, Mulrain L, Navidi A, Naylor J, Negash T, Nguyen T, Nguyen N, Nicol R, Norbu C, Norbu N, Novod N, O'Neill B, Osman S, Markiewicz E, Oyono OL, Patti C, Phunkhang P, Pierre F, Priest M, Raghuraman S, Rege F, Reyes R, Rise C, Rogov P, Ross K, Ryan E, Settipalli S, Shea T, Sherpa N, Shi L, Shih D, Sparrow T, Spaulding J, Stalker J, Stange-Thomann N, Stavropoulos S, Stone C, Strader C, Tesfaye S, Thomson T, Thoulutsang Y, Thoulutsang D, Topham K, Topping I, Tsamla T, Vassiliev H, Vo A, Wangchuk T, Wangdi T, Weiand M, Wilkinson J, Wilson A, Yadav S, Young G, Yu Q, Zembek L, Zhong D, Zimmer A, Zwirko Z, Alvarez P, Brockman W, Butler J, Chin C, Grabherr M, Kleber M, Mauceli E, MacCallum I. Evolution of genes and genomes on the Drosophila phylogeny. Nature. 2007;450:203–218. - PubMed
Publication types
MeSH terms
Substances
Grants and funding
LinkOut - more resources
Full Text Sources
Molecular Biology Databases
Research Materials