Ebola virus entry requires the host-programmed recognition of an intracellular receptor - PubMed
- ️Sun Jan 01 2012
. 2012 Apr 18;31(8):1947-60.
doi: 10.1038/emboj.2012.53. Epub 2012 Mar 6.
Gregor Obernosterer, Matthijs Raaben, Andrew S Herbert, Maika S Deffieu, Anuja Krishnan, Esther Ndungo, Rohini G Sandesara, Jan E Carette, Ana I Kuehne, Gordon Ruthel, Suzanne R Pfeffer, John M Dye, Sean P Whelan, Thijn R Brummelkamp, Kartik Chandran
Affiliations
- PMID: 22395071
- PMCID: PMC3343336
- DOI: 10.1038/emboj.2012.53
Ebola virus entry requires the host-programmed recognition of an intracellular receptor
Emily Happy Miller et al. EMBO J. 2012.
Abstract
Ebola and Marburg filoviruses cause deadly outbreaks of haemorrhagic fever. Despite considerable efforts, no essential cellular receptors for filovirus entry have been identified. We showed previously that Niemann-Pick C1 (NPC1), a lysosomal cholesterol transporter, is required for filovirus entry. Here, we demonstrate that NPC1 is a critical filovirus receptor. Human NPC1 fulfills a cardinal property of viral receptors: it confers susceptibility to filovirus infection when expressed in non-permissive reptilian cells. The second luminal domain of NPC1 binds directly and specifically to the viral glycoprotein, GP, and a synthetic single-pass membrane protein containing this domain has viral receptor activity. Purified NPC1 binds only to a cleaved form of GP that is generated within cells during entry, and only viruses containing cleaved GP can utilize a receptor retargeted to the cell surface. Our findings support a model in which GP cleavage by endosomal cysteine proteases unmasks the binding site for NPC1, and GP-NPC1 engagement within lysosomes promotes a late step in entry proximal to viral escape into the host cytoplasm. NPC1 is the first known viral receptor that recognizes its ligand within an intracellular compartment and not at the plasma membrane.
Conflict of interest statement
Brummelkamp, Carette, Chandran, Raaben, and Whelan are co-inventors on a patent application that describes NPC1 as a target for development of antiviral therapies against filoviruses.
Figures
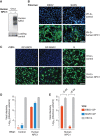
Human NPC1 confers susceptibility to filovirus entry and infection. (A) Viper VH-2 cells were engineered to express empty vector or human NPC1. Expression of human NPC1 was determined by immunoblotting (IB) with an anti-NPC1 antibody. Proteins non-specifically detected by this antibody were used as a loading control. Samples for IB of NPC1 were resolved on one gel and loading controls were resolved on another. (B, C) Infection of control and NPC1-expressing VH-2 cells by wild-type filoviruses (B), or recombinant VSVs bearing VSV or filovirus glycoproteins (C). Infected cells (green) and counter-stained nuclei (blue) were visualized by fluorescence microscopy. Scale bars, 20 μm. (D, E) Infectivity of VSV pseudotypes bearing VSV or filovirus glycoproteins in control and NPC1-expressing VH-2 cells. (E) NPC1-expressing VH-2 cells were pretreated with the cysteine protease inhibitor E-64 (300 μM) for 4 h at 37°C and then exposed to virus. (D, E) Results (n=3) are representative of two independent experiments. Error bars indicate s.d. Asterisk indicates values below the limit of detection. Figure source data can be found in Supplementary data.
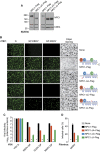
NPC1 luminal loop domain C is required for filovirus entry, but full-length NPC1 is dispensable. (A) NPC1-null CHO CT43 cells were engineered to express mutant forms of human NPC1–Flag lacking domains A, C, or I. NPC1 expression was determined by IB with an anti-Flag antibody. Cyclin-dependent kinase 4 (CDK4) in each sample was detected by IB (Abcam) and provided a loading control. Samples for IB of each NPC1 mutant and its paired WT NPC1 control were resolved on the same gel. NPC1 and CDK4 were detected on separate gels. (B) Capacity of mutant NPC1 proteins to rescue viral entry and transport lysosomal cholesterol. (Left) Infection of NPC1-null CHO CT43 cells expressing mutant NPC1–Flag proteins by recombinant VSVs bearing VSV G or filovirus glycoproteins. Infected cells (green) were visualized by fluorescence microscopy. (Right) Cholesterol clearance by mutant NPC1–Flag proteins in CT43 cells was determined by filipin staining and fluorescence microscopy. Images were inverted for clarity. Scale bars, 20 μm. (C, D) Infectivity of VSV pseudotypes bearing VSV or filovirus glycoproteins (C) and wild-type MARV (D) in CT43 cells expressing mutant NPC–Flag proteins. SUDV, Sudan virus. Results in (C) (n=4–6) are from two independent experiments. Results in (D) (n=3) are from a representative experiment. Error bars indicate s.d. Asterisks indicate values below the limit of detection. Figure source data can be found in Supplementary data.
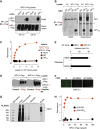
NPC1 binds specifically and directly to a cleaved form of the Ebola virus glycoprotein. (A) Co-immunoprecipitation (co-IP) of NPC1 by EBOV GP. Magnetic beads coated with GP-specific monoclonal antibody KZ52 were incubated with detergent extracts containing no virus (None), uncleaved rVSV-GP, or cleaved rVSV-GPCL. The resulting control or glycoprotein-decorated beads were mixed with cell lysates containing human NPC1–Flag at pH 7.5 or 5.1 and 4°C. Beads were then retrieved and NPC1–Flag in the immune pellets and supernatants was detected by IB with an anti-Flag antibody. Pellets and supernatants were resolved on separate gels but exposed simultaneously to the same piece of film. (B) Reciprocal co-IP of GP by NPC1. Cell lysates lacking (Ctrl) or containing NPC1–Flag were incubated with anti-Flag antibody-coated magnetic beads. The resulting control or NPC1-decorated beads were mixed with detergent extracts of rVSV-GP or rVSV-GPCL at pH 7.5 and 4°C. Beads were then retrieved and GP or GPCL in the immune pellets and supernatants was detected by IB with an anti-GP antiserum. Pellets and supernatants were resolved on separate gels but exposed simultaneously to the same piece of film. Asterisks indicate bands detected non-specifically by the antiserum. (C) GPCL captures NPC1 but not NPC1-like1 (NPC1L1) in an ELISA. Plates coated with rVSV-GP or rVSV-GPCL were incubated with cell extracts containing NPC1–Flag or NPC1L1–Flag, and bound Flag-tagged proteins were detected with an anti-Flag antibody. Results (n=3) are representative of at least four independent experiments. (D) Cell extracts used in (C) were incubated with plates coated with an anti-Flag antibody or an isotype-matched control, and captured proteins were eluted and detected by IB with the anti-Flag antibody. Samples were resolved on the same gel. (E, F) NPC1L1 cannot support filovirus entry and infection. CT43 cells expressing NPC1L1–Flag were exposed to wild-type EBOV or MARV (E) or to recombinant VSVs (F), and infected cells were visualized and enumerated by fluorescence microscopy. Asterisks in (E) indicate values below the limit of detection. Scale bar, 20 μm. (G, H) GPCL but not GP captures affinity-purified NPC1–Flag in an ELISA. (G) NPC1–Flag was purified from CT43 CHO cell lysates by Flag affinity chromatography and visualized by SDS–PAGE and staining with Krypton infrared protein stain. Samples were resolved on the same gel. (H) ELISA plates coated with rVSV-GP or rVSV-GPCL were incubated with NPC1–Flag purified in (G), and bound Flag-tagged proteins were detected with an anti-Flag antibody. Results (n=3) are representative of at least four independent experiments. Error bars indicate s.d. Figure source data can be found in Supplementary data.
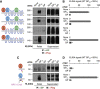
NPC1 luminal domain C is necessary and sufficient for GP–NPC1 binding. (A, B) Binding of NPC1 mutants lacking domains A, C, or I to EBOV GP. (A) Co-IP of mutant NPC1–Flag proteins from CT43 cell extracts by rVSV-GP was carried out as described in Figure 3A. Pellet samples for each NPC1 construct were resolved on the same gel. Pellets and supernatants were resolved on separate gels but exposed simultaneously to the same piece of film. (B) Capture of mutant NPC1–Flag proteins by rVSV-GP in an ELISA was carried out as described in Figure 3C. (C, D) CT43 cells were engineered to express synthetic single-pass membrane proteins comprising individual luminal domains of NPC1 fused to the first transmembrane domain of NPC1, the NPC1 cytoplasmic tail, and a Flag tag. Binding of these NPC1 mutants to EBOV GP was determined by co-IP (C) and ELISA (D) as described above. Results in (B) and (D) (n=3) are representative of at least three independent experiments. Error bars indicate s.d. Figure source data can be found in Supplementary data.
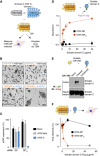
Soluble forms of NPC1 domain C bind directly to GP and selectively neutralize infection by viral particles containing cleaved glycoproteins. (A–C) Capacity of an rVSV displaying a domain C-VSV G chimera (rVSV-domain C) to bind to rVSV-GP and neutralize rVSV-GP infection. (A) Schematic of experiments shown in (A–C). ‘rVSV-bald’ particles lack any virus-encoded glycoproteins. (B) rVSV-GP and rVSV-GPCL were preincubated with rVSV-domain C or rVSV-bald, and virus mixtures were stained with phosphotungstic acid and visualized by electron microscopy. Arrows indicate large clusters of viral particles. A larger version of this image is available in Supplementary Figure S5. (C) Virus mixtures were exposed to WT CHO cells, and expression of the rVSV-GP-encoded eGFP gene was quantitated at 7 h post infection (see Materials and methods for details). eGFP signal was normalized to that of uncleaved virus. (D) The capacity of rVSV-GP and rVSV-GPCL to capture a purified, soluble form of domain C containing Flag and hexahistidine tags was determined in an ELISA, as described in Figure 3C. Results (n=3) are representative of at least three independent experiments. (E) The capacity of a purified, soluble form of GP lacking the transmembrane domain (GPΔTM) to associate with purified, soluble domain C was determined by co-IP, as described in Figure 3A. Pellets and supernatants (one gel for each) were resolved on separate gels but exposed simultaneously to the same piece of film. (F) rVSV-GP and rVSV-GPCL were preincubated with soluble domain C, and virus–protein mixtures were exposed to the Vero African grivet monkey kidney cell line. Viral infection was enumerated by fluorescence microscopy. Results are from two independent experiments (n=4). Error bars indicate s.d. Figure source data can be found in Supplementary data.
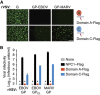
A synthetic single-pass membrane protein containing domain C can mediate filovirus entry. CT43 cells expressing synthetic membrane proteins containing individual NPC1 luminal domains (see Figure 4C) were exposed to rVSVs bearing uncleaved or cleaved filovirus glycoproteins. Infected cells (green) were visualized (A) and enumerated (B) by fluorescence microscopy. Results in (B) (n=3) are representative of at least three independent experiments. Error bars indicate s.d. Asterisks in (B) indicate values below the limit of detection. Scale bar in (A), 20 μm.
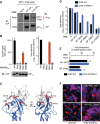
Binding of NPC1 to a site within the Ebola virus glycoprotein is required at a late step in entry proximal to viral escape into the cytoplasm. (A) Co-IP of NPC1 by cleaved VSV-GPCL particles containing EBOV GP(WT) or GP(3Ala) carrying three point mutations in the proposed GP receptor-binding site (see text for details). NPC1–Flag and GP in immune pellets were detected by IB with an anti-Flag antibody and an anti-GP antiserum, respectively. Samples for IB of NPC1 and GP were resolved on separate gels (one gel for detection of each protein). (B) (Left) Capture of NPC1–Flag proteins by VSV-GPCL containing WT or mutant GPs in an ELISA. An IB of input cleaved viral particles is shown below the graph. Samples were resolved on the same gel. Equivalent amounts of each GP bound to the prefusion conformation-specific antibody KZ52 were used in the NPC1-capture ELISA. (Right) Infectivity of WT and mutant VSV-GP in WT CHO cells. Results (n=3) are representative of two independent experiments. (C) Cartoon representations of the putative NPC1-binding site in GP1 (PDB id: 3CSY; Lee et al, 2008). Blue, head subdomain; grey, glycan cap subdomain. Mutated residues are shown as red ball-and-sticks. (D) Infectivity of VSV-GP bearing WT or mutant GPs in control and NPC1-overexpressing WT CHO cells. GP(F535R) contains a mutation in the GP2 fusion loop that inhibits membrane insertion (Supplementary Figure S9). Asterisk indicates values below the limit of detection. Results (n=3) are representative of at least two independent experiments. (E) Attachment of eGFP-containing virus-like particles (VLPs) bearing GP(WT) or GP(3Ala) to control and NPC1-overexpressing WT CHO cells. VLPs were exposed to cells at 4°C for 30 min and binding was quantitated by flow cytometry. Percent of eGFP-positive cells was determined by gating on the eGFP signal (see Supplementary Figure S8). Results (n=4–5) are from two independent experiments. (F) Viral escape into the cytoplasm was measured by release of VSV M protein derived from entering VSV-GPCL. Control and NPC1-overexpressing WT CHO cells were exposed to VSV-GPCL containing GP(WT) or GP(3Ala) (multiplicity of infection, MOI=200) for 3 h and processed for VSV M staining. VSV M (red) and counter-stained nuclei (blue) were visualized by fluorescence microscopy. Scale bar, 20 μm. Figure source data can be found in Supplementary data.
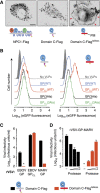
Cleaved but not uncleaved filovirus glycoproteins can utilize a synthetic cell surface receptor based on NPC1 domain C for viral entry. (A) Distribution of NPC1 in CT43 cells expressing WT NPC1–Flag, loop C-Flag, and loop C-Flagtailless (lacking the NPC1 cytoplasmic tail). Cells were immunostained with an anti-Flag antibody and visualized by fluorescence microscopy. Red arrowheads indicate signal at the cell surface. Images were inverted for clarity. The capacity of each protein to localize to the plasma membrane (PM) (grey, no localization to PM; red, localization to PM) is indicated in the cartoon below each image. Scale bar, 10 μm. (B) Attachment of VLPs containing uncleaved or cleaved GP(WT) or GP(3Ala) to CT43 cells expressing domain C-Flag or domain C-Flagtailless. VLPs were exposed to cells at 4°C for 30 min and binding was determined by flow cytometry. (C) Infectivity of rVSV-GP-EBOV, EBOV rVSV-GPCL-EBOV, and rVSV-GP-MARV in CT43 cells expressing domain C-Flag or domain C-Flagtailless. Results (n=4) are from two independent experiments. (D) rVSV-GP-MARV was allowed to attach to CT43 cells expressing domain C-Flag or domain C-Flagtailless at 4°C for 45 min, and cells were treated with 0 (−), 50, 100, or 150 μg/ml thermolysin at 37°C for 5 min. Cells were then washed to remove residual protease and unbound virus, and viral infectivity was determined at 16 h post infection. Asterisks indicate values below the limit of detection. Results (n=4) are from two independent experiments. Error bars indicate s.d.
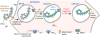
A model for the role of NPC1 in Ebola virus and Marburg virus entry. Virus particles adhere to the plasma membrane by binding to one or more cell attachment factors. They are then trafficked to early/late endosomal (EE/LE) compartments where host cysteine proteases, including cathepsin B (Chandran et al, 2005), cleave GP to remove the mucin and glycan cap domains and expose the NPC1-binding site. This cleaved GP species, which resembles in vitro-cleaved GPCL, can then bind to NPC1's domain C, presumably on the limiting membrane of an LE or lysosomal (Lys) compartment. GP–NPC1 interaction may play a direct role in triggering GP conformational changes that bring about viral membrane fusion and cytoplasmic release of the viral nucleocapsid payload. The molecular requirements for, and intracellular location of, this final step in filovirus entry remain to be defined.
Similar articles
-
Mittler E, Alkutkar T, Jangra RK, Chandran K. Mittler E, et al. mBio. 2021 Jan 12;12(1):e03100-20. doi: 10.1128/mBio.03100-20. mBio. 2021. PMID: 33436438 Free PMC article.
-
Bornholdt ZA, Ndungo E, Fusco ML, Bale S, Flyak AI, Crowe JE Jr, Chandran K, Saphire EO. Bornholdt ZA, et al. mBio. 2016 Feb 23;7(1):e02154-15. doi: 10.1128/mBio.02154-15. mBio. 2016. PMID: 26908579 Free PMC article.
-
Ebola virus entry requires the cholesterol transporter Niemann-Pick C1.
Carette JE, Raaben M, Wong AC, Herbert AS, Obernosterer G, Mulherkar N, Kuehne AI, Kranzusch PJ, Griffin AM, Ruthel G, Dal Cin P, Dye JM, Whelan SP, Chandran K, Brummelkamp TR. Carette JE, et al. Nature. 2011 Aug 24;477(7364):340-3. doi: 10.1038/nature10348. Nature. 2011. PMID: 21866103 Free PMC article.
-
Filovirus entry: a novelty in the viral fusion world.
Hunt CL, Lennemann NJ, Maury W. Hunt CL, et al. Viruses. 2012 Feb;4(2):258-75. doi: 10.3390/v4020258. Epub 2012 Feb 7. Viruses. 2012. PMID: 22470835 Free PMC article. Review.
-
Emerging intracellular receptors for hemorrhagic fever viruses.
Jae LT, Brummelkamp TR. Jae LT, et al. Trends Microbiol. 2015 Jul;23(7):392-400. doi: 10.1016/j.tim.2015.04.006. Epub 2015 May 21. Trends Microbiol. 2015. PMID: 26004032 Review.
Cited by
-
Martin B, Hoenen T, Canard B, Decroly E. Martin B, et al. Antiviral Res. 2016 Nov;135:1-14. doi: 10.1016/j.antiviral.2016.09.001. Epub 2016 Sep 14. Antiviral Res. 2016. PMID: 27640102 Free PMC article. Review.
-
Mingo RM, Simmons JA, Shoemaker CJ, Nelson EA, Schornberg KL, D'Souza RS, Casanova JE, White JM. Mingo RM, et al. J Virol. 2015 Mar;89(5):2931-43. doi: 10.1128/JVI.03398-14. Epub 2014 Dec 31. J Virol. 2015. PMID: 25552710 Free PMC article.
-
Host cell factors in filovirus entry: novel players, new insights.
Hofmann-Winkler H, Kaup F, Pöhlmann S. Hofmann-Winkler H, et al. Viruses. 2012 Dec;4(12):3336-62. doi: 10.3390/v4123336. Viruses. 2012. PMID: 23342362 Free PMC article. Review.
-
Poirier S, Mayer G, Murphy SR, Garver WS, Chang TY, Schu P, Seidah NG. Poirier S, et al. Traffic. 2013 Apr;14(4):458-69. doi: 10.1111/tra.12046. Epub 2013 Feb 20. Traffic. 2013. PMID: 23350547 Free PMC article.
-
A Single Residue in Ebola Virus Receptor NPC1 Influences Cellular Host Range in Reptiles.
Ndungo E, Herbert AS, Raaben M, Obernosterer G, Biswas R, Miller EH, Wirchnianski AS, Carette JE, Brummelkamp TR, Whelan SP, Dye JM, Chandran K. Ndungo E, et al. mSphere. 2016 Mar 30;1(2):e00007-16. doi: 10.1128/mSphere.00007-16. eCollection 2016 Mar-Apr. mSphere. 2016. PMID: 27303731 Free PMC article.
References
-
- Becker S, Spiess M, Klenk HD (1995) The asialoglycoprotein receptor is a potential liver-specific receptor for Marburg virus. J Gen Virol 76(Part 2): 393–399 - PubMed
-
- Berger AC, Salazar G, Styers ML, Newell-Litwa KA, Werner E, Maue RA, Corbett AH, Faundez V (2007) The subcellular localization of the Niemann-Pick Type C proteins depends on the adaptor complex AP-3. J Cell Sci 120: 3640–3652 - PubMed
Publication types
MeSH terms
Substances
Grants and funding
LinkOut - more resources
Full Text Sources
Other Literature Sources
Medical