Persisting viral sequences shape microbial CRISPR-based immunity - PubMed
- ️Invalid Date
Persisting viral sequences shape microbial CRISPR-based immunity
Ariel D Weinberger et al. PLoS Comput Biol. 2012.
Abstract
Well-studied innate immune systems exist throughout bacteria and archaea, but a more recently discovered genomic locus may offer prokaryotes surprising immunological adaptability. Mediated by a cassette-like genomic locus termed Clustered Regularly Interspaced Short Palindromic Repeats (CRISPR), the microbial adaptive immune system differs from its eukaryotic immune analogues by incorporating new immunities unidirectionally. CRISPR thus stores genomically recoverable timelines of virus-host coevolution in natural organisms refractory to laboratory cultivation. Here we combined a population genetic mathematical model of CRISPR-virus coevolution with six years of metagenomic sequencing to link the recoverable genomic dynamics of CRISPR loci to the unknown population dynamics of virus and host in natural communities. Metagenomic reconstructions in an acid-mine drainage system document CRISPR loci conserving ancestral immune elements to the base-pair across thousands of microbial generations. This 'trailer-end conservation' occurs despite rapid viral mutation and despite rapid prokaryotic genomic deletion. The trailer-ends of many reconstructed CRISPR loci are also largely identical across a population. 'Trailer-end clonality' occurs despite predictions of host immunological diversity due to negative frequency dependent selection (kill the winner dynamics). Statistical clustering and model simulations explain this lack of diversity by capturing rapid selective sweeps by highly immune CRISPR lineages. Potentially explaining 'trailer-end conservation,' we record the first example of a viral bloom overwhelming a CRISPR system. The polyclonal viruses bloom even though they share sequences previously targeted by host CRISPR loci. Simulations show how increasing random genomic deletions in CRISPR loci purges immunological controls on long-lived viral sequences, allowing polyclonal viruses to bloom and depressing host fitness. Our results thus link documented patterns of genomic conservation in CRISPR loci to an evolutionary advantage against persistent viruses. By maintaining old immunities, selection may be tuning CRISPR-mediated immunity against viruses reemerging from lysogeny or migration.
Conflict of interest statement
The authors have declared that no competing interests exist.
Figures
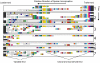
Metagenomic reconstructions of the first CRISPR locus of a G-plasma population sampled in 2002 (1), 2005 (2), June 2006 (3), August 2006 (4) November 2006 (5), May 2007 (6) and August 2007 (7). In each sampling, the CRISPR spacers (boxes) are aligned horizontally according to their ordering in the metagenomic reads, with CRISPR repeats removed for compactness. Overlapping 454 spacer patterns are also condensed (Methods). The left-ends are the leader-ends, where new spacers are unidirectionally incorporated. Boxes filled with the same color represent identical spacers, with two exceptions. Black-filled boxes show flanking genetic material and white-filled boxes denote cell-specific spacers found only once in the dataset. White gaps reflect unsequenced regions in the metagenomic reconstructions. When separated spacers can be linked via paired reads, the intervening region is shown as a grey bar. Boxes containing a black ‘X’ indicate probable spacer deletions. When spacers match reconstructed AMDV3b viral sequences, diamonds are inserted, with filled diamonds showing perfect matches and open diamonds reflecting imperfect matches. Trailer-end conservation (presumed immunological memory) and clonality are pronounced in this locus, with large numbers of matching spacers preserved across the six-year period. Another example of trailer-end conservation and clonality—in the CRISPR loci of archaeal I-plasma—is shown in Figure S1.
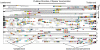
Metagenomic reconstructions of the second CRISPR locus of G-plasma at the seven sampled time points. Notably, several trailer-end G-plasma spacers match reconstructed AMDV3b across all time points (filled diamonds).
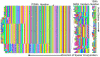
Computational reconstructions show the loss of trailer-end diversity from CRISPR loci. Reconstructions show the 45 most frequent host strains at the 100th, 500th and 7000th iterations of a representative simulation without spacer deletion. In each panel, the rows show distinct host strains, with their spacers allayed across the columns from right to left as in Figures 1, 2 and S1. Circles indicate spacers perfectly matching any of the 300 most frequent viral strains in that iteration. To preserve space, 128 clonal columns are removed in iteration 7000 prior to the divergence of sub-populations from a common ancestor (arrow). Notably one ancestral population still at low frequency (∼0.007 as shown in Figure S4) in the 100th iteration is the common ancestor of all surviving strains.
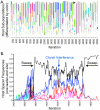
(A) Hosts CRISPR loci from the simulation in Figure 3 are clustered (Text S3) into distinct sub-populations every 100 model iterations to capture how trailer-end clonality emerges. Cluster heights represent the cumulative frequencies of all strains in a given cluster, cluster widths show the number of distinct strains in that cluster, and the combined height of all clusters in an iteration reflects the fraction of virus-host interactions that is immune (i.e., host mean fitness). A marked loss of host diversity occurs prior to iteration 3800 (→), after which the sweeping sub-population diversifies through distinct leader-end spacer incorporations (Figure S6). (B) The frequencies of all host spacers at a single leader-end column are tracked during the clustering-predicted sweep. A single spacer (shown in black) rapidly rises in frequency before iteration 3800 as predicted by the clustering. Subsequent ‘kill the winner’ oscillations occur before all competing hosts go extinct. A second sweep purges the remaining diversity at this locus position.
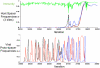
In the upper panel, the frequency of the sweeping spacer identified in Figure 4B is again shown in black. Also tracked, are the two adjacent spacers added by the black spacer's successful host line. The frequencies of these adjacent spacers in their respective locus columns are shown in red and blue. In green, we track the fraction of immune virus-host interactions. The lower panel shows the frequencies of the three corresponding proto-spacers in the viral population. The inverse fluctuations in viral proto-spacer frequencies show that the viruses fail to lose all three proto-spacers on a single line until just prior to iteration 3800, after the sweep. The host line thus sweeps due to immunity to both viral sub-populations.
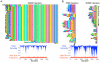
The model is extended to allow a parameterized fraction of (single) spacer additions in host CRISPR loci to occur with deletions of randomly-sized blocks of spacers from random locus positions. The lower panels in (A) and (B) plot host immunity (blue) against maximum viral strain frequency (red) in each iteration. (A) When 5% of additions occur with deletions, trailer-end memory and clonality are preserved. Only new-end spacers target current viruses and CRISPR's antiviral immunity is maintained at high levels across thousands of model iterations. (B) When 50% of spacer additions occur with deletions, trailer-end memory and clonality are purged. Depletions in host immunity occur (lower panel), indicating viral blooms due to the large fraction of interactions in which CRISPR fails to provide immunity (i.e., host and virus do not share spacers). During the predicted bloom at iteration 768, immunity against the top 300 viral strains is conferred by two older spacers, which are lost from most host lines prior to the bloom (Figure S8).
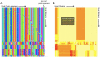
(A) The 651 viruses at the model predicted bloom (iteration 768 in Figure 6B) are shown along the rows, with the virus' 50 aligned protospacers shown along the columns. Distinct proto-spacers are colored differently. Strains are then clustered based on proto-spacer relatedness. The two proto-spacers providing immunity at the bloom (Figures 6B and S8), are shifted to the two rightmost columns for clarity. Five distinct viral sub-populations are observed in the mosaic, with the largest blooming sub-population characterized by closely related mutants sharing the two critical proto-spacers in their rightmost columns. (B) Viral and host sub-populations at the bloom are superimposed on one another to reveal ‘cloud on cloud’ immunity at the bloom. The rows contain viral strains and the columns show host strains. Each entry of the heat-mapped ‘immunity matrix’ shows the number of shared spacers between the respective host and viral strain. Pale yellow color represents no shared spacers (susceptibility), yellow one shared spacer, orange two shared spacers, and red three shared spacers. The silhouette width (Text S3) was maximized to cluster both hosts (columns) and viruses (rows) into an optimal number of sub-populations based on immunity profiles. Distinct host sub-populations possess immunity to distinct viral sub-populations.
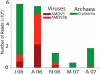
Number of sequencing reads of G-plasma and its viral populations, AMDV3 and AMDV3b, calculated from the community genomic data at a single location across five time points in 2006–2007. Relative abundances of all archaeal, bacterial, viral and plasmid genomes reconstructed from this community during 2006–2007 are shown in Figure S9. Both Figures 8 and S9, capture a bloom of AMDV3b virus (bright red) at the second time point, August 2006, coincident with the depletion of its archaeal G-plasma host (bright green). Notably, the G-plasma CRISPR loci from these time points were reconstructed in samplings (3)–(7) of Figures 1 and 2. G-plasma contained several spacers exactly matching the blooming AMDV3b sequences prior to the August 2006 bloom (black diamonds in Figure 2).
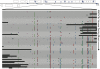
Sequence variation within a gene of the blooming AMDV3b viral population (345 bp field of view). The top bar of the figure represents an 18 kb contig sequence of AMDV3b, with predicted genes shown as boxes. Below the contig, is a close-up view of sequence variation within a single gene. White bars represent aligned sequencing reads, while colored bars indicate SNPs relative to the composite sequence. The black region is a large deleted sequence block in one individual viral genome. Distinct viral sub-populations are captured during the bloom, each sharing common SNPs. Also shown in the figure are regions of the AMDV3b contig that match G-plasma spacers: closed circles in the contig represent perfect matches and open circles represent imperfect matches. When the match between G-plasma spacer and AMDV3b protospacer occurs within a predicted gene, the circle is placed inside the corresponding gene box; matches to intergenic regions are shown below on the contig line.
Similar articles
-
Viral diversity threshold for adaptive immunity in prokaryotes.
Weinberger AD, Wolf YI, Lobkovsky AE, Gilmore MS, Koonin EV. Weinberger AD, et al. mBio. 2012 Dec 4;3(6):e00456-12. doi: 10.1128/mBio.00456-12. mBio. 2012. PMID: 23221803 Free PMC article.
-
Childs LM, Held NL, Young MJ, Whitaker RJ, Weitz JS. Childs LM, et al. Evolution. 2012 Jul;66(7):2015-29. doi: 10.1111/j.1558-5646.2012.01595.x. Epub 2012 Mar 19. Evolution. 2012. PMID: 22759281 Free PMC article.
-
Pseudo-chaotic oscillations in CRISPR-virus coevolution predicted by bifurcation analysis.
Berezovskaya FS, Wolf YI, Koonin EV, Karev GP. Berezovskaya FS, et al. Biol Direct. 2014 Jul 2;9:13. doi: 10.1186/1745-6150-9-13. Biol Direct. 2014. PMID: 24986220 Free PMC article.
-
Al-Attar S, Westra ER, van der Oost J, Brouns SJ. Al-Attar S, et al. Biol Chem. 2011 Apr;392(4):277-89. doi: 10.1515/BC.2011.042. Epub 2011 Feb 7. Biol Chem. 2011. PMID: 21294681 Review.
-
Koonin EV, Wolf YI. Koonin EV, et al. Mol Biosyst. 2015 Jan;11(1):20-7. doi: 10.1039/c4mb00438h. Epub 2014 Sep 19. Mol Biosyst. 2015. PMID: 25238531 Free PMC article. Review.
Cited by
-
Polyclonality of concurrent natural populations of Alteromonas macleodii.
Gonzaga A, Martin-Cuadrado AB, López-Pérez M, Megumi Mizuno C, García-Heredia I, Kimes NE, Lopez-García P, Moreira D, Ussery D, Zaballos M, Ghai R, Rodriguez-Valera F. Gonzaga A, et al. Genome Biol Evol. 2012;4(12):1360-74. doi: 10.1093/gbe/evs112. Genome Biol Evol. 2012. PMID: 23212172 Free PMC article.
-
Probabilistic models for CRISPR spacer content evolution.
Kupczok A, Bollback JP. Kupczok A, et al. BMC Evol Biol. 2013 Feb 26;13:54. doi: 10.1186/1471-2148-13-54. BMC Evol Biol. 2013. PMID: 23442002 Free PMC article.
-
Global transcription of CRISPR loci in the human oral cavity.
Lum AG, Ly M, Santiago-Rodriguez TM, Naidu M, Boehm TK, Pride DT. Lum AG, et al. BMC Genomics. 2015 May 21;16(1):401. doi: 10.1186/s12864-015-1615-0. BMC Genomics. 2015. PMID: 25994215 Free PMC article.
-
Bernheim A, Bikard D, Touchon M, Rocha EPC. Bernheim A, et al. Nucleic Acids Res. 2020 Jan 24;48(2):748-760. doi: 10.1093/nar/gkz1091. Nucleic Acids Res. 2020. PMID: 31745554 Free PMC article.
-
Programmable plasmid interference by the CRISPR-Cas system in Thermococcus kodakarensis.
Elmore JR, Yokooji Y, Sato T, Olson S, Glover CV 3rd, Graveley BR, Atomi H, Terns RM, Terns MP. Elmore JR, et al. RNA Biol. 2013 May;10(5):828-40. doi: 10.4161/rna.24084. Epub 2013 Mar 27. RNA Biol. 2013. PMID: 23535213 Free PMC article.
References
-
- Labrie SJ, Samson JE, Moineau S. Bacteriophage resistance mechanisms. Nat Rev Microbiol. 2010;8:317–327. - PubMed
-
- Wilson GG, Murray NE. Restriction and modification systems. Annu Rev Genet. 1991;25:585–627. - PubMed
-
- Edwards RA, Rohwer F. Viral metagenomics. Nat Rev Microbiol. 2005;3:504–510. - PubMed
-
- Barrangou R, Fremaux C, Deveau H, Richards M, Boyaval P, et al. CRISPR provides acquired resistance against viruses in prokaryotes. Science. 2007;315:1709–1712. - PubMed
Publication types
MeSH terms
LinkOut - more resources
Full Text Sources
Other Literature Sources