Trypanosomal immune evasion, chronicity and transmission: an elegant balancing act - PubMed
- ️Sun Jan 01 2012
Review
Trypanosomal immune evasion, chronicity and transmission: an elegant balancing act
Paula MacGregor et al. Nat Rev Microbiol. 2012.
Abstract
During their life cycle, trypanosomes must overcome conflicting demands to ensure their survival and transmission. First, they must evade immunity without overwhelming the host. Second, they must generate and maintain transmission stages at sufficient levels to allow passage into their tsetse vector. Finally, they must rapidly commit to onward development when they enter the tsetse fly. On the basis of recent quantification and modelling of Trypanosoma brucei infection dynamics, we propose that the interplay between immune evasion and development achieves both infection chronicity and transmissibility. Moreover, we suggest that a novel form of bistable regulation ensures developmental commitment on entry into the tsetse fly midgut.
Figures
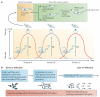
a | African trypanosomes are extracellular parasites that survive through their capacity for antigenic variation. During an infection, a procession of distinct antigen types emerges, each controlled by the host immune response. Simplistically, this generates an undulating parasitaemia. In each wave of parasitaemia, proliferative slender forms are responsible for the increase in parasite numbers. This is accompanied by the accumulation of a parasite-derived factor, stumpy induction factor (SIF), that stimulates slender cells to transform to stumpy forms. Stumpy forms are non-proliferative, being arrested in G0–G1, and are the transmission stage of the parasite, capable of developing further when ingested by the tsetse fly during a blood meal. Stumpy cells are irreversibly committed to cell cycle exit in the bloodstream and are removed from the population by a combination of immune clearance and cell ageing. When stumpy forms are taken up by a tsetse fly, they differentiate to midgut procyclic forms; slender forms that are taken up are killed. The procyclic forms proliferate before arresting and migrating to the salivary glands, where they attach as short epimastigote forms. These develop to metacyclic forms, which are unattached to the salivary gland wall and infective to mammals. b | During a trypanosomal infection in mammalian hosts, the probability of expression for variant surface glycoprotein (VSG) genes is governed by the activation mechanism of each gene. Early in the infection, in situ switches allow telomeric VSG genes that are encoded in expression sites to be activated. Subsequently, intact VSG genes that are encoded in subtelomeric regions are expressed. Later in infection, mosaic VSG genes can contribute to the repertoire. These are assembled from incomplete VSG genes, and their activation can be dependent on the previously active VSG gene (as they are created through homologous recombination), resulting in a ‘string’ of related VSG genes being expressed.
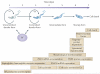
Uncommitted slender cells release and receive stumpy induction factor (SIF), stimulating them to begin the transition to stumpy forms. Mathematical modelling predicts that, initially, cells develop to a committed slender form that is capable of up to three rounds of cell division before cell cycle exit in G1, whereupon SIF production is lost. The resulting intermediate forms no longer express the haptoglobin-haemoglobin receptor but express mRNAs encoding PAD1 (proteins associated with differentiation 1) and ESAG9 proteins. The morphological transformation to stumpy forms involves the production of a broader cell form with a prominent, undulating membrane and the development of the mitochondrion and of diaphorase activity. As stumpy forms mature, their flagellar pocket increases in size and the lysosome is repositioned. In some cases, the nucleus moves to the cell posterior, displacing the terminal kinetoplast and generating posteronuclear stumpy forms. Stumpy cells are eventually removed from the bloodstream; mathematical modelling predicts a life expectancy of 55 hours, in agreement with experimental observations of 48-72 hours.
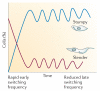
Early in infection, slender cells dominate and undergo rapid switching in their variant surface glycoproteins (VSGs), assisting the establishment of the parasite population in a host that has been exposed to earlier infections or has a current infection. When the parasite population is established, it becomes predominantly stumpy, ensuring the transmissibility of the parasite (as stumpy forms are optimized for uptake by the tsetse fly vector). The small number of slender forms that are still present undergo VSG switching at a high rate and thus continue to feed new antigenic variants into the bloodstream to maintain a chronic infection.
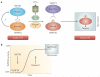
a | In stumpy forms of trypanosomes, differentiation is prevented by the activity of trypanosomal phosphotyrosine phosphatase 1 (TbPTP1), which dephosphorylates (and so represses) the serine/threonine-specific phosphatase TbPIP39. The interaction between TbPIP39 and TbPTP1 stimulates the activity of TbPTP1, thus reinforcing the inactivation of TbPIP39 and generating a stable OFF state. In the presence of CCA (citrate and/or cis-aconitate), the stimulation of TbPTP1 is lost, potentially through steric interference between TbPIP39 and TbPTP1 (generated by the presence of a TbPIP39 citrate-binding pocket). This favours the phosphorylation (and so activation) of TbPIP39 by an unknown kinase (kinase X). Both active and inactive TbPIP39 are unidirectionally trafficked to the glycosome, where active TbPIP39 dephosphorylates unidentified glycosomal targets. As phosphorylated TbPIP39 is locked in the glycosomes, it cannot be dephosphorylated by TbPTP1, thus ensuring its irreversible activation. This generates a stable ON state. b | The activity of TbPIP39 in response to the actions of TbPTP1 and kinase X. TbPIP39 is activated by phosphorylation, but this is rendered irreversible by the compartmentalization of the enzyme in the glycosome. PAD, proteins associated with differentiation.
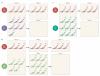
a | Cells that produce and respond to stumpy induction factor (SIF) (P+R+ cells) generate transmissible stumpy forms and are maintained throughout multiple host–vector transactions. If cells no longer respond to SIF (P+R− cells), they gain a short-term advantage because they outgrow the P+R+ cells (which respond to SIF and therefore exit the cell cycle). However, because these P+R− cells produce less transmissible forms, their long-term viability is lower. b | Cells that can respond to the SIF produced by others in the population but do not produce SIF themselves (P−R+ cells) would have a growth advantage if the production of SIF were costly. This could promote their transmission to a new host. However, in this new host the absence of SIF benefactors would reduce the number of transmission stages and so limit the long-term viability of these cells. c | For cells that neither produce nor respond to SIF (P−R− cells), there would be a short-term advantage, as they would continue to proliferate and would out-grow other cells (including P+R−cells) because they do not incur the costs associated with SIF production. However, their transmission potential would be greatly reduced owing to the absence of stumpy forms, selecting against them in the long term.
Similar articles
-
Matetovici I, Caljon G, Van Den Abbeele J. Matetovici I, et al. BMC Genomics. 2016 Nov 25;17(1):971. doi: 10.1186/s12864-016-3283-0. BMC Genomics. 2016. PMID: 27884110 Free PMC article.
-
Trypanosoma brucei: infectivity and immunogenicity of cultured parasites.
Nyindo M, Wellde BT. Nyindo M, et al. Exp Parasitol. 1985 Oct;60(2):150-4. doi: 10.1016/0014-4894(85)90017-7. Exp Parasitol. 1985. PMID: 4029345
-
Dewar CE, Casas-Sanchez A, Dieme C, Crouzols A, Haines LR, Acosta-Serrano Á, Rotureau B, Schnaufer A. Dewar CE, et al. mBio. 2022 Feb 22;13(1):e0235721. doi: 10.1128/mbio.02357-21. Epub 2022 Jan 11. mBio. 2022. PMID: 35012336 Free PMC article.
-
Bloodstream form pre-adaptation to the tsetse fly in Trypanosoma brucei.
Rico E, Rojas F, Mony BM, Szoor B, Macgregor P, Matthews KR. Rico E, et al. Front Cell Infect Microbiol. 2013 Nov 14;3:78. doi: 10.3389/fcimb.2013.00078. eCollection 2013. Front Cell Infect Microbiol. 2013. PMID: 24294594 Free PMC article. Review.
-
Flying tryps: survival and maturation of trypanosomes in tsetse flies.
Dyer NA, Rose C, Ejeh NO, Acosta-Serrano A. Dyer NA, et al. Trends Parasitol. 2013 Apr;29(4):188-96. doi: 10.1016/j.pt.2013.02.003. Epub 2013 Mar 16. Trends Parasitol. 2013. PMID: 23507033 Review.
Cited by
-
Evolutionary cell biology of chromosome segregation: insights from trypanosomes.
Akiyoshi B, Gull K. Akiyoshi B, et al. Open Biol. 2013 May 1;3(5):130023. doi: 10.1098/rsob.130023. Open Biol. 2013. PMID: 23635522 Free PMC article. Review.
-
Trypanosoma brucei Parasites Occupy and Functionally Adapt to the Adipose Tissue in Mice.
Trindade S, Rijo-Ferreira F, Carvalho T, Pinto-Neves D, Guegan F, Aresta-Branco F, Bento F, Young SA, Pinto A, Van Den Abbeele J, Ribeiro RM, Dias S, Smith TK, Figueiredo LM. Trindade S, et al. Cell Host Microbe. 2016 Jun 8;19(6):837-48. doi: 10.1016/j.chom.2016.05.002. Epub 2016 May 26. Cell Host Microbe. 2016. PMID: 27237364 Free PMC article.
-
Shimogawa MM, Saada EA, Vashisht AA, Barshop WD, Wohlschlegel JA, Hill KL. Shimogawa MM, et al. Mol Cell Proteomics. 2015 Jul;14(7):1977-88. doi: 10.1074/mcp.M114.045146. Epub 2015 May 11. Mol Cell Proteomics. 2015. PMID: 25963835 Free PMC article.
-
Stoking the drug target pipeline for human African trypanosomiasis.
Phillips MA. Phillips MA. Mol Microbiol. 2012 Oct;86(1):10-4. doi: 10.1111/mmi.12001. Epub 2012 Aug 28. Mol Microbiol. 2012. PMID: 22925123 Free PMC article.
-
Genome-wide dissection of the quorum sensing signalling pathway in Trypanosoma brucei.
Mony BM, MacGregor P, Ivens A, Rojas F, Cowton A, Young J, Horn D, Matthews K. Mony BM, et al. Nature. 2014 Jan 30;505(7485):681-685. doi: 10.1038/nature12864. Epub 2013 Dec 15. Nature. 2014. PMID: 24336212 Free PMC article.
References
-
- Barrett MP. The rise and fall of sleeping sickness. Lancet. 2006;367:1377–1378. - PubMed
-
- Brun R, Blum J, Chappuis F, Burri C. Human African trypanosomiasis. Lancet. 2010;375:148–159. - PubMed
-
- Vassella E, Reuner B, Yutzy B, Boshart M. Differentiation of African trypanosomes is controlled by a density sensing mechanism which signals cell cycle arrest via the cAMP pathway. J. Cell Sci. 1997;110:2661–2671. - PubMed
-
- Reuner B, Vassella E, Yutzy B, Boshart M. Cell density triggers slender to stumpy differentiation of Trypanosoma brucei bloodstream forms in culture. Mol. Biochem. Parasitol. 1997;90:269–280. - PubMed
-
- Roditi I, Lehane MJ. Interactions between trypanosomes and tsetse flies. Curr. Opin. Microbiol. 2008;11:345–351. - PubMed
Publication types
MeSH terms
LinkOut - more resources
Full Text Sources
Medical