The NSL complex regulates housekeeping genes in Drosophila - PubMed
- ️Invalid Date
The NSL complex regulates housekeeping genes in Drosophila
Kin Chung Lam et al. PLoS Genet. 2012.
Abstract
MOF is the major histone H4 lysine 16-specific (H4K16) acetyltransferase in mammals and Drosophila. In flies, it is involved in the regulation of X-chromosomal and autosomal genes as part of the MSL and the NSL complexes, respectively. While the function of the MSL complex as a dosage compensation regulator is fairly well understood, the role of the NSL complex in gene regulation is still poorly characterized. Here we report a comprehensive ChIP-seq analysis of four NSL complex members (NSL1, NSL3, MBD-R2, and MCRS2) throughout the Drosophila melanogaster genome. Strikingly, the majority (85.5%) of NSL-bound genes are constitutively expressed across different cell types. We find that an increased abundance of the histone modifications H4K16ac, H3K4me2, H3K4me3, and H3K9ac in gene promoter regions is characteristic of NSL-targeted genes. Furthermore, we show that these genes have a well-defined nucleosome free region and broad transcription initiation patterns. Finally, by performing ChIP-seq analyses of RNA polymerase II (Pol II) in NSL1- and NSL3-depleted cells, we demonstrate that both NSL proteins are required for efficient recruitment of Pol II to NSL target gene promoters. The observed Pol II reduction coincides with compromised binding of TBP and TFIIB to target promoters, indicating that the NSL complex is required for optimal recruitment of the pre-initiation complex on target genes. Moreover, genes that undergo the most dramatic loss of Pol II upon NSL knockdowns tend to be enriched in DNA Replication-related Element (DRE). Taken together, our findings show that the MOF-containing NSL complex acts as a major regulator of housekeeping genes in flies by modulating initiation of Pol II transcription.
Conflict of interest statement
The authors have declared that no competing interests exist.
Figures
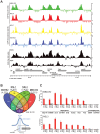
(A) Genome Browser snapshot of a gene-rich region on chromosome 3 L. The log2FCs (ChIP/input) of the newly generated ChIP-seq data of MBD-R2 and NSL3 are compared to those of NSL1, MCRS2 , WDS (GEO: GSE20835) and MOF (GEO: GSE27806). (B) The Venn diagram of NSL-bound TSS regions reveals an extensive set of promoters that are concomitantly bound by all four NSL proteins. As indicated in the cartoon below the Venn diagram, a promoter was called NSL-bound if the 400 bp region surrounding the TSS (gray dashed lines) overlapped with the summit region of a peak determined by MACS and PeakSplitter (dashed blue lines). Using this definition, we identified a total of 6,510 TSSs bound by at least one NSL protein and 2,841 bound by all four. The numbers below the ChIP-ed protein names indicate the numbers of bound TSSs. (C) Chromatin immunoprecipitation followed by quantitative real-time PCR for a set of NSL target genes (Bap170, CG6506, sec5, CG15011, Ent2, Incenp, tho2, Patj) and non-target genes (ODSH, CG14872) confirm the results of the genome-wide ChIP-seq analyses: NSL proteins predominantly bind to the 5′ end of genes. Primers were designed to target the promoter (Pro), middle (Mid) and end (End) of genes; error bars represent standard deviations obtained from three independent experiments.
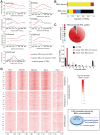
(A) Metagene profiles of histone modifications reveal higher ratios of active chromatin marks H3K4me2/3, H4K16ac and H3K9ac for active genes bound by the NSL complex compared to active NSL-non-bound and inactive genes. On the contrary, the repressive mark H3K27me2 is not enriched on gene promoters bound by the NSL complex. Active genes were defined according to the expression data from (see Materials and Methods). The expression levels of NSL-bound and NSL-non-bound active genes are similar (Figure S2A). The log2 ratios ( = log2FC (ChIP/input)) of the histone modifications were obtained from modENCODE, extracted for 200 bp bins, and normalized to H4 Chip-chip signals. (B) The chromatin color model contains two states of euchromatin: “yellow” and “red”. NSL-bound TSSs are predominantly associated with “yellow”, but not “red” chromatin. NSL-non-bound genes display chromatin color ratios that resemble the pattern seen by Filion et al. for tissue-specific genes. (“Green” and “blue” correspond to classical and PcG heterochromatin, respectively, while “black” denotes regions of repressive chromatin). (C) For a different model of chromatin states devised by Kharchenko et al., similar results as in Figure 2B were obtained: The pie chart depicts that 93% of all peaks of NSL1, MCRS2, NSL3 and MBD-R2 that localize within +/−200 bp of the nearest TSS associate with regions of chromatin state 1. This is defined as the state of actively transcribed TSSs . Complementary, as shown in the bar chart, NSL-bound TSSs of expressed genes are significantly enriched in chromatin state 1 and depleted of chromatin state 9 (p-values<2.2e-16; binomial test) while NSL-non-bound genes are more equally distributed between states of active TSSs (1) and elongation marks (states 2, 3, 4). (D) Heatmaps of ChIP-seq signals (log2FCs) demonstrate the strong enrichment of NSL binding around the TSSs of constitutively transcribed genes. In contrast to the Pol II signal that is present in both constitutive and regulatory (not constitutive) active genes, the NSL proteins are predominantly found around the TSSs of constitutively transcribed genes. As indicated on the left-hand side, genes were sorted according to their genomic location. The proteins' binding intensities can be directly compared between the different panels. The inlay (right) illustrates the findings of the heatmap with a focus on genes that are bound by all NSLs concomitantly: 85.5% of NSL-bound promoters are constitutively expressed (light blue area). Active (not constitutive) and inactive genes are represented by dark blue and white areas, respectively.
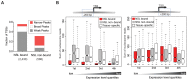
(A) The TSSs of constitutively active genes, either NSL-bound or –non-bound, were analyzed regarding their reported transcription initiation patterns . NSL-bound TSSs mostly belong to genes with weak and broad transcription initiation peaks (40% and 11.4%) whereas NSL-non-bound TSSs mainly belong to genes with narrow transcription initiation peaks (19.3%). (B) Boxplots of the sum of overlapping nucleosome reads in the regions 200 bp upstream and 200 bp downstream of the TSSs of constitutively expressed NSL-bound genes (red), constitutively expressed NSL-non-bound genes (gray), and tissue-specific genes (white). Genes were stratified based on their gene expression quartile (see Materials and Methods) which demonstrates that the depletion of nucleosomes immediately upstream of the TSS that we observed for NSL-bound housekeeping genes (left side) is independent of expression levels (p-values for −200 bp region <2.2e-16; Wilcoxon test).
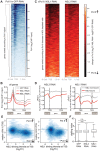
(A) The heatmap displays input-normalized Pol II binding signals for 5′ ends of D. melanogaster genes as captured by ChIP-seq of Rbp3 in S2 cells that had been treated with dsRNA against GFP. Genes were sorted according to the signal strength: Genes with high Pol II binding on promoters as well as along the gene bodies are found in the upper part of the heatmap. They are followed by genes with Pol II binding primarily at the promoter and genes lacking detectable Pol II signals. (B) Metagene profiles of the genome-wide signals of Pol II shows a marked decrease of Pol II binding for cells lacking NSL1 or NSL3 compared to control cells. (C) Here, the change of Pol II binding upon knockdown of NSL1 and NSL3 (ΔPol II) was visualized. The ΔPol II signal is calculated as the difference of normalized Pol II ChIP-seq signal (log2FC) in NSL-depleted cells and control cells. Genes are ranked according to the change of Pol II in NSL knockdown; genes with greatest Pol II loss are found at the top of the heatmap. Severe reduction of Pol II after NSL depletion is seen around the TSSs and along gene bodies (dark red to black color), but there are also numerous genes that are slightly or not affected (bright red color). (D) Average ΔPol II values were plotted for active genes, separated into NSL-bound and –non-bound ones. The general decrease of Pol II upon NSL knockdown was observed again. In addition, it now becomes more evident that the magnitude of Pol II loss is markedly higher in NSL-bound genes compared to NSL-non-bound genes. (E) To study the association between the loss of Pol II (i.e. negative ΔPol II values) and NSL binding in an unbiased manner, median ΔPol II values at promoters were plotted against the median binding intensities of NSL1 and NSL3 from wild type samples. Genes were filtered for non-overlapping genes and those with significant Pol II binding in the control sample; the promoter region was defined as a 400 bp region centered around the TSS. The scatter plots confirm that genes with substantial NSL signals show markedly lower ΔPol II values than genes without NSL binding (left hand side of the plot). The difference of ΔPol II between NSL-bound and NSL-non-bound genes is statistically highly significant as determined by Wilcoxon rank sum test (p-value<2.2e-16). The observation that the majority of the genes with high NSL binding display a negative ΔPol II value (Pol II loss), suggests the NSL complex as a transcriptional activator whose binding to genes has functional consequences. (F) Stalling indexes for all genes with significant Pol II binding in control and NSL-depleted cells were calculated. Stalling indexes are derived from the ratio of Pol II at the promoter versus Pol II along the gene body (see Materials and Methods); high stalling indexes indicate Pol II accumulation at the promoter and diminished release into transcriptional elongation. No statistically significant difference between the stalling indexes of genes in the three different conditions was observed (median stalling indexes are 1.611 for GFP-RNAi treated cells, 1.649 in NSL3-RNAi treated cells and 1.848 in NSL1-RNAi, p-value>0.1, Wilcoxon rank sum test; n.s. = not significant).
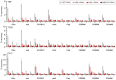
ChIP was performed with antibodies against TBP, TFIIB and Pol II (Rpb3) in NSL1, NSL3 and MBD-R2 depleted S2 cells as well as in GFP knockdown control cells. The quantitative qPCR was performed on six autosomal genes (P5cr, ns4, CG15011, tho2, Patj, CG5098) as well as 2 X-linked genes (CG6506 and CG4406). Primers were positioned at the promoter (P), middle (M) and end (E) of the indicated genes. Percentage recovery is determined as the amount of immunoprecipitated DNA relative to input DNA. Error bars represent the standard deviation between independent experiments.
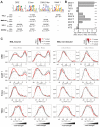
(A) Individual de novo motif analysis led to the discovery of four non-repetitive DNA motifs that are located within NSL-complex binding sites (r.c. = reverse complement). The analysis was carried out by MEME , for 100 bp regions around the peak summits. As computational restrictions of MEME allowed only a limited number of base pairs to be analyzed at a time, the results of the 500 highest peak regions are shown here (for additional peak regions see Figure S7). (B) Motif enrichments were calculated with TRAP , using the motif matrices for the 10 known core promoter motifs identified by . In our study, the TRAP score can be seen as a measure for the affinity of transcription factors to bind to the DNA regions of interest. We compared the TRAP scores for NSL-bound and –non-bound promoter regions (TSS +/−200 bp) and found Ohler motifs 1, 6, 7, 8 as well as DRE and E-box significantly and selectively enriched in NSL-target regions while TATA box, Inr, DPE and MTE are depleted. The bar plot depicts the fold change between the median TRAP scores of NSL-bound versus –non-bound regions; individual frequency distributions of the motifs' TRAP scores can be seen in Figure S8 (for constitutitve gene promoters). P-values for the comparison of NSL-bound versus –non-bound promoters were calculated with two-sided Wilcoxon rank sum test, **** = P<0.0001, *** = P<0.001, ** = P<0.01, * = P<0.5. (C) To determine the significance of the Ohler motifs for the function of the NSL complex, genes were divided into three classes according to the magnitude of Pol II loss. The 1st subset (red line) corresponds to genes with the most severe Pol II reduction upon NSL knockdown while the 3rd subset (light gray line) contains least affected genes. Density distributions of TRAP scores were then plotted for NSL-bound and –non-bound genes for each Ohler motif individually. For DRE and motif 1 there is a clear distinction between the differently affected NSL-bound genes: NSL targets that lose Pol II binding most dramatically after NSL knockdown (red line) are clearly enriched for high DRE TRAP scores. In contrast, motif 1 shows an inverse pattern compared to DRE: NSL-bound genes with mild Pol II loss (light gray line) tend to contain strong motif 1 sites. This trend is not observed in NSL-non-bound genes. Other motifs such as E-box and TATA box also did not show significant association (also see Figure S9).
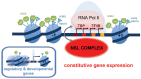
The majority of the NSL-bound targets are constitutively expressed or “housekeeping” genes. These genes are characterized by prominent enrichment of particular histone modifications (H4K16ac, H3K9ac, H3K4me2, H3K4me3) as well as specific core promoter elements (such as DRE, E-box and motif 1; indicated by colored squares). In contrast, tissue-specific or developmentally regulated genes (small inlay) usually contain the TATA-box as the most prominent core promoter element. We propose that the NSL complex acts as a regulator of constitutively expressed genes by facilitating stable recruitment of the pre-initiation complex (PIC) members such as Pol II, TBP and TFIIB on target genes. NSL complex may therefore serve as an important link between specific promoter architecture and PIC assembly.
Similar articles
-
The nonspecific lethal complex is a transcriptional regulator in Drosophila.
Raja SJ, Charapitsa I, Conrad T, Vaquerizas JM, Gebhardt P, Holz H, Kadlec J, Fraterman S, Luscombe NM, Akhtar A. Raja SJ, et al. Mol Cell. 2010 Jun 25;38(6):827-41. doi: 10.1016/j.molcel.2010.05.021. Mol Cell. 2010. PMID: 20620954
-
Zhao X, Su J, Wang F, Liu D, Ding J, Yang Y, Conaway JW, Conaway RC, Cao L, Wu D, Wu M, Cai Y, Jin J. Zhao X, et al. PLoS Genet. 2013 Nov;9(11):e1003940. doi: 10.1371/journal.pgen.1003940. Epub 2013 Nov 14. PLoS Genet. 2013. PMID: 24244196 Free PMC article.
-
Schiemann AH, Li F, Weake VM, Belikoff EJ, Klemmer KC, Moore SA, Scott MJ. Schiemann AH, et al. BMC Mol Biol. 2010 Nov 9;11:80. doi: 10.1186/1471-2199-11-80. BMC Mol Biol. 2010. PMID: 21062452 Free PMC article.
-
Hung KH, Stumph WE. Hung KH, et al. Crit Rev Biochem Mol Biol. 2011 Feb;46(1):11-26. doi: 10.3109/10409238.2010.518136. Epub 2010 Oct 6. Crit Rev Biochem Mol Biol. 2011. PMID: 20925482 Review.
-
Getting up to speed with transcription elongation by RNA polymerase II.
Jonkers I, Lis JT. Jonkers I, et al. Nat Rev Mol Cell Biol. 2015 Mar;16(3):167-77. doi: 10.1038/nrm3953. Epub 2015 Feb 18. Nat Rev Mol Cell Biol. 2015. PMID: 25693130 Free PMC article. Review.
Cited by
-
Kudron M, Gevirtzman L, Victorsen A, Lear BC, Gao J, Xu J, Samanta S, Frink E, Tran-Pearson A, Huynh C, Vafeados D, Hammonds A, Fisher W, Wall M, Wesseling G, Hernandez V, Lin Z, Kasparian M, White K, Allada R, Gerstein M, Hillier L, Celniker SE, Reinke V, Waterston RH. Kudron M, et al. bioRxiv [Preprint]. 2024 Jan 20:2024.01.18.576242. doi: 10.1101/2024.01.18.576242. bioRxiv. 2024. PMID: 38293065 Free PMC article. Updated. Preprint.
-
Gaub A, Sheikh BN, Basilicata MF, Vincent M, Nizon M, Colson C, Bird MJ, Bradner JE, Thevenon J, Boutros M, Akhtar A. Gaub A, et al. Nat Commun. 2020 May 7;11(1):2243. doi: 10.1038/s41467-020-16103-0. Nat Commun. 2020. PMID: 32382029 Free PMC article.
-
Dai A, Wang Y, Greenberg A, Liufu Z, Tang T. Dai A, et al. Front Genet. 2021 Jun 14;12:675027. doi: 10.3389/fgene.2021.675027. eCollection 2021. Front Genet. 2021. PMID: 34194473 Free PMC article.
-
Huang CJ, Lyu X, Kang J. Huang CJ, et al. Cell Cycle. 2023 Mar-Mar;22(6):619-632. doi: 10.1080/15384101.2022.2145816. Epub 2022 Nov 16. Cell Cycle. 2023. PMID: 36384428 Free PMC article. Review.
-
An H4K16 histone acetyltransferase mediates decondensation of the X chromosome in C. elegans males.
Lau AC, Zhu KP, Brouhard EA, Davis MB, Csankovszki G. Lau AC, et al. Epigenetics Chromatin. 2016 Oct 19;9:44. doi: 10.1186/s13072-016-0097-x. eCollection 2016. Epigenetics Chromatin. 2016. PMID: 27777629 Free PMC article.
References
-
- Kouzarides T. Chromatin modifications and their function. Cell. 2007;128:693–705. - PubMed
-
- Yang XJ, Seto E. HATs and HDACs: from structure, function and regulation to novel strategies for therapy and prevention. Oncogene. 2007;26:5310–5318. - PubMed
-
- Jacobson RH, Ladurner AG, King DS, Tjian R. Structure and function of a human TAFII250 double bromodomain module. Science. 2000;288:1422–1425. - PubMed
Publication types
MeSH terms
Substances
LinkOut - more resources
Full Text Sources
Molecular Biology Databases