Exome sequencing identifies recurrent somatic RAC1 mutations in melanoma - PubMed
. 2012 Sep;44(9):1006-14.
doi: 10.1038/ng.2359. Epub 2012 Jul 29.
Yong Kong, Byung Hak Ha, Perry Evans, Antonella Bacchiocchi, James P McCusker, Elaine Cheng, Matthew J Davis, Gerald Goh, Murim Choi, Stephan Ariyan, Deepak Narayan, Ken Dutton-Regester, Ana Capatana, Edna C Holman, Marcus Bosenberg, Mario Sznol, Harriet M Kluger, Douglas E Brash, David F Stern, Miguel A Materin, Roger S Lo, Shrikant Mane, Shuangge Ma, Kenneth K Kidd, Nicholas K Hayward, Richard P Lifton, Joseph Schlessinger, Titus J Boggon, Ruth Halaban
Affiliations
- PMID: 22842228
- PMCID: PMC3432702
- DOI: 10.1038/ng.2359
Exome sequencing identifies recurrent somatic RAC1 mutations in melanoma
Michael Krauthammer et al. Nat Genet. 2012 Sep.
Abstract
We characterized the mutational landscape of melanoma, the form of skin cancer with the highest mortality rate, by sequencing the exomes of 147 melanomas. Sun-exposed melanomas had markedly more ultraviolet (UV)-like C>T somatic mutations compared to sun-shielded acral, mucosal and uveal melanomas. Among the newly identified cancer genes was PPP6C, encoding a serine/threonine phosphatase, which harbored mutations that clustered in the active site in 12% of sun-exposed melanomas, exclusively in tumors with mutations in BRAF or NRAS. Notably, we identified a recurrent UV-signature, an activating mutation in RAC1 in 9.2% of sun-exposed melanomas. This activating mutation, the third most frequent in our cohort of sun-exposed melanoma after those of BRAF and NRAS, changes Pro29 to serine (RAC1(P29S)) in the highly conserved switch I domain. Crystal structures, and biochemical and functional studies of RAC1(P29S) showed that the alteration releases the conformational restraint conferred by the conserved proline, causes an increased binding of the protein to downstream effectors, and promotes melanocyte proliferation and migration. These findings raise the possibility that pharmacological inhibition of downstream effectors of RAC1 signaling could be of therapeutic benefit.
Conflict of interest statement
COMPETING FINANCIAL INTERESTS
The authors declare no competing financial interests.
Figures
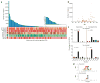
Mutation spectrum in melanoma samples. (a) Numbers of somatic nonsynonymous mutations across 99 matched melanoma samples. The tumors are represented as originating from sun-exposed skin (dark orange bars) or sun-shielded skin (acral (A), mucosal (M) or uveal (U), shown in different shades of light orange) or are melanomas of unknown origin (gray bars). Melanomas from primary compared to metastasis, the age of the patients and BRAF and RAS mutation status are also indicated. Mut, mutated; WT, wild type. NRAS is the most common RAS mutant gene, except for one acral melanoma that harbored the HRAS mutation resulting in p.Gln61His (brown). All BRAF and RAS mutations were validated by Sanger sequencing. The inset shows an expanded scale of the number of mutations in tumors at the lower range. (b) The number of mutations in relation to the age of the patients. (c) Spectrum of somatic variants in exomes of sun-exposed and sun-shielded (acral, mucosal and uveal) melanomas. There is an excess of C>T transitions in dipyrimidines in sun-exposed melanomas, which is an indication of UV exposure and DNA damage. The n values indicate the number of mutations; because any base has a 25% chance of being flanked by a purine on both sides, the frequency at which a mutated base is part of a dipyrimidine by chance is exactly threefold the frequency for nondipyrimidines. (d) Consensus sequence for positions with recurrent (at least three) and nonrecurrent mutations, as indicated. The recurrent mutations are located at a known hotspot sequence motif for cyclobutane pyrimidine dimer formation. The motif represents the actual prevalence of each sequence context without normalizing for the mammalian under-representation of CG dinucleotides.
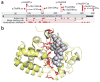
Schematic representation of PPP6C alterations. (a) Map of PPP6C functional domains and the sites of amino acid substitutions. The horizontal bar shows the 342 amino acids of PPP6C (NP_001116827.1), the light gray bar shows the serine/threonine phosphatase domain, and the red peaks indicate the active site, the metal-binding sites and the heterodimer interface downloaded from NCBI. The red circles indicate the 11 mutations we discovered in the complete melanoma cohort and validated as somatic by Sanger sequencing. Recurrent mutations are indicated by multiple circles. (b) Mutant residues in PPP6C mapped onto the crystal structure of the phosphatase domain of PP2A (Protein Data Bank (PDB) 2IE4). All but one of the amino acids found to be mutated are conserved between PPP6C and PP2A. The PP2A structure is in complex with tumor-inducing okadaic acid, shown as solid spheres, which binds to the catalytic cleft. Mutations discovered in melanoma are shown in red. There is a clear preference for melanoma mutations to occur in the catalytic cleft of PPP6C.
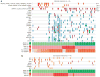
Distribution of SCNAs and genes with high mutation loads in melanomas. (a) A plot showing copy number aberrations and high somatic nonsynonymous mutation load. Red and brown indicate copy gain and loss, respectively, as related to chromosome band (right) and focal gene in the region (left). Mutations are marked in blue, and mutations occurring at LOH are shown in purple. At the bottom, the melanoma subtypes described in Figure 1a are indicated. (b) A plot of genes with a high load of somatic nonsense mutations. The bars indicate point mutations (orange), indels (pink) and splice-site variants (purple) that lead to frame shifts and early chain termination.
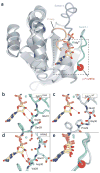
Crystal structure of RAC1P29S. (a) Overall schematic of RAC1P29S showing the P-loop (pink), switch I (light green), switch II (purple), Mg2+ (cyan) and the slowly hydrolyzing GTP analog, GMP-PNP (stick format). The red sphere indicates the location of p.Pro29Ser. The dashed box indicates the regions shown in b–e. (b) Close-up view showing close contacts of ribose hydroxyls with the switch I region in RAC1P29S. (c) RAC1WT bound to GMP-PNP. (d) HRAS in complex with GMP-PNP (PDB 5P21). Direct hydrogen bonding between the switch I backbone and ribose are commonly observed in activated HRAS but are less frequently observed in Rho family GTPases, including RAC1. A RAS-like hydrogen bonding pattern is observed in activated RAC1P29S (dashed red lines). (e) Superposition of the switch I region and GMP-PNP. RAC1P29S is shown in light green, RAC1WT is shown in gray, and HRAS is shown in light blue. The red sphere indicates the location of p.Pro29Ser. The residues discussed in the text are labeled. The figure was made using CCP4mg.
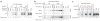
In vitro RAC1P29S binding to downstream effectors. (a) PAK1 pulldown assay showing that recombinant histidine-tagged RAC1P29S (His-RAC1P29S) has higher binding to its effector PAK1 compared to His-RAC1WT for samples that include GTP and GTPγS. NC, PAK1-PBD beads alone as a negative control. The His-RAC1WT and His-RACP29S lanes show unbound wild-type and mutant protein, as indicated. The multiple bands in all lanes are RAC1; all bands are observed when RAC1 crystals are run on SDS-PAGE. (b) RAC1 pulldown assay showing that recombinant His-GST-RAC1P29S has higher binding to endogenous MLK3 expressed in melanoma cells compared to His-GST-RAC1WT in all reaction samples. The immobilized recombinant RAC1 proteins were incubated with cell lysates from two independent melanomas (YULAC that is RAC1WT and BRAFV600K and YUHEF that is RAC1P29S and BRAFWT). The figure shows western blot of bound proteins with antibodies to MLK3. As negative controls, we used lysates incubated with GST-bound beads or recombinant RAC1 proteins that were not incubated with cell extracts. The western blot analysis also shows the expression of the relevant proteins in whole-cell lysate (WCL). The reaction mixtures were likely to contain nucleotides contributed by the cell lysates in addition to those added in vitro, as indicated. (c) PAK1 pulldown assay comparing the binding activity of RAC1WT, RAC1P29S and RAC1F28L proteins. The numbers under each lane indicate the band density as determined by scanning the membranes with ImageJ.
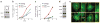
Cellular function of RAC1P29S compared to RAC1WT. (a) Western blot analysis of RAC1 in mouse melanocytes infected with wild-type (WT) or mutant RACP29S retroviral expression vectors compared to nontransfected cells (parental). Actin indicates protein loading in each lane. (b) RAC1P29S enhances melanocyte proliferation. The proliferation of melanocytes transiently expressing RAC1WT (green) or RAC1P29S (red) compared to noninfected parental cells (blue). Error bars indicate standard errors. (c) RAC1P29S enhances cell migration. The graphs show the rate of migration of parental, RAC1WT- and RAC1P29S-expressing melanocytes at daily intervals. (d) RAC1P29S enhances ERK activation. Western blots of lysates from mouse melanocytes expressing RAC1WT or RAC1P29S probed with RAC1, pERK, ERK and actin antibodies, as indicated on the side of the gel. (e) Localization of GFP-tagged RAC1WT (GFP-RAC1WT) and GFP-RAC1P29S in COS-7 cells. COS-7 cells transiently expressing GFP-RAC1WT (i, ii) or GFP-RAC1P29S (iii, iv) were fixed with paraformaldehyde and imaged with a spinning-disk confocal-based inverted Olympus microscope. Insets (yellow boxes) illustrate the localization of RAC1P29S, but not RAC1WT, in membrane ruffles (compare i and ii to the yellow arrows in iii and iv, respectively). pERK, phosphorylated ERK. Scale bars, 10 μm.
Similar articles
-
Mar VJ, Wong SQ, Logan A, Nguyen T, Cebon J, Kelly JW, Wolfe R, Dobrovic A, McLean C, McArthur GA. Mar VJ, et al. Pigment Cell Melanoma Res. 2014 Nov;27(6):1117-25. doi: 10.1111/pcmr.12295. Epub 2014 Aug 4. Pigment Cell Melanoma Res. 2014. PMID: 25043693
-
Characterisation and outcome of RAC1 mutated melanoma.
Lodde GC, Jansen P, Herbst R, Terheyden P, Utikal J, Pföhler C, Ulrich J, Kreuter A, Mohr P, Gutzmer R, Meier F, Dippel E, Weichenthal M, Sucker A, Placke JM, Zaremba A, Albrecht LJ, Kowall B, Galetzka W, Becker JC, Tasdogan A, Zimmer L, Livingstone E, Hadaschik E, Schadendorf D, Ugurel S, Griewank K. Lodde GC, et al. Eur J Cancer. 2023 Apr;183:1-10. doi: 10.1016/j.ejca.2023.01.009. Epub 2023 Jan 18. Eur J Cancer. 2023. PMID: 36773463
-
Mutational status of naevus-associated melanomas.
Shitara D, Tell-Martí G, Badenas C, Enokihara MM, Alós L, Larque AB, Michalany N, Puig-Butille JA, Carrera C, Malvehy J, Puig S, Bagatin E. Shitara D, et al. Br J Dermatol. 2015 Sep;173(3):671-80. doi: 10.1111/bjd.13829. Epub 2015 Jun 19. Br J Dermatol. 2015. PMID: 25857817 Free PMC article.
-
Somatic driver mutations in melanoma.
Reddy BY, Miller DM, Tsao H. Reddy BY, et al. Cancer. 2017 Jun 1;123(S11):2104-2117. doi: 10.1002/cncr.30593. Cancer. 2017. PMID: 28543693 Review.
-
Recent advances in cutaneous melanoma: towards a molecular model and targeted treatment.
Read J. Read J. Australas J Dermatol. 2013 Aug;54(3):163-72. doi: 10.1111/ajd.12013. Epub 2013 Jan 18. Australas J Dermatol. 2013. PMID: 23330781 Review.
Cited by
-
From melanocytes to melanomas.
Shain AH, Bastian BC. Shain AH, et al. Nat Rev Cancer. 2016 Jun;16(6):345-58. doi: 10.1038/nrc.2016.37. Epub 2016 Apr 29. Nat Rev Cancer. 2016. PMID: 27125352 Review.
-
mTORC1 activation blocks BrafV600E-induced growth arrest but is insufficient for melanoma formation.
Damsky W, Micevic G, Meeth K, Muthusamy V, Curley DP, Santhanakrishnan M, Erdelyi I, Platt JT, Huang L, Theodosakis N, Zaidi MR, Tighe S, Davies MA, Dankort D, McMahon M, Merlino G, Bardeesy N, Bosenberg M. Damsky W, et al. Cancer Cell. 2015 Jan 12;27(1):41-56. doi: 10.1016/j.ccell.2014.11.014. Cancer Cell. 2015. PMID: 25584893 Free PMC article.
-
Melanoma cells homing to the brain: an in vitro model.
Rizzo A, Vasco C, Girgenti V, Fugnanesi V, Calatozzolo C, Canazza A, Salmaggi A, Rivoltini L, Morbin M, Ciusani E. Rizzo A, et al. Biomed Res Int. 2015;2015:476069. doi: 10.1155/2015/476069. Epub 2015 Jan 26. Biomed Res Int. 2015. PMID: 25692137 Free PMC article.
-
The P-Rex1/Rac signaling pathway as a point of convergence for HER/ErbB receptor and GPCR responses.
Kazanietz MG, Barrio-Real L, Casado-Medrano V, Baker MJ, Lopez-Haber C. Kazanietz MG, et al. Small GTPases. 2018 Jul 4;9(4):297-303. doi: 10.1080/21541248.2016.1221273. Epub 2016 Sep 2. Small GTPases. 2018. PMID: 27588611 Free PMC article. Review.
-
Approaches of targeting Rho GTPases in cancer drug discovery.
Lin Y, Zheng Y. Lin Y, et al. Expert Opin Drug Discov. 2015;10(9):991-1010. doi: 10.1517/17460441.2015.1058775. Epub 2015 Jun 18. Expert Opin Drug Discov. 2015. PMID: 26087073 Free PMC article. Review.
References
-
- Ribas A, Flaherty KT. BRAF targeted therapy changes the treatment paradigm in melanoma. Nat Rev Clin Oncol. 2011;8:426–433. - PubMed
Publication types
MeSH terms
Substances
LinkOut - more resources
Full Text Sources
Other Literature Sources
Medical
Research Materials
Miscellaneous