Large-scale introgression shapes the evolution of the mating-type chromosomes of the filamentous ascomycete Neurospora tetrasperma - PubMed
- ️Wed Oct 23 2148
Large-scale introgression shapes the evolution of the mating-type chromosomes of the filamentous ascomycete Neurospora tetrasperma
Yu Sun et al. PLoS Genet. 2012.
Abstract
The significance of introgression as an evolutionary force shaping natural populations is well established, especially in animal and plant systems. However, the abundance and size of introgression tracts, and to what degree interspecific gene flow is the result of adaptive processes, are largely unknown. In this study, we present medium coverage genomic data from species of the filamentous ascomycete Neurospora, and we use comparative genomics to investigate the introgression landscape at the genomic level in this model genus. We revealed one large introgression tract in each of the three investigated phylogenetic lineages of Neurospora tetrasperma (sizes of 5.6 Mbp, 5.2 Mbp, and 4.1 Mbp, respectively). The tract is located on the chromosome containing the locus conferring sexual identity, the mating-type (mat) chromosome. The region of introgression is confined to the region of suppressed recombination and is found on one of the two mat chromosomes (mat a). We used Bayesian concordance analyses to exclude incomplete lineage sorting as the cause for the observed pattern, and multilocus genealogies from additional species of Neurospora show that the introgression likely originates from two closely related, freely recombining, heterothallic species (N. hispaniola and N. crassa/N. perkinsii). Finally, we investigated patterns of molecular evolution of the mat chromosome in Neurospora, and we show that introgression is correlated with reduced level of molecular degeneration, consistent with a shorter time of recombination suppression. The chromosome specific (mat) and allele specific (mat a) introgression reported herein comprise the largest introgression tracts reported to date from natural populations. Furthermore, our data contradicts theoretical predictions that introgression should be less likely on sex-determining chromosomes. Taken together, the data presented herein advance our general understanding of introgression as a force shaping eukaryotic genomes.
Conflict of interest statement
The authors have declared that no competing interests exist.
Figures
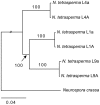
The tree was generated by maximum likelihood analysis of 1,978 concatenated autosomal genes. Bootstrap value from 100 replicates is shown by each branch.
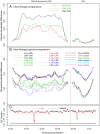
The pair-wise divergences were calculated as the fraction of differences (in bp) between the sequences, using a sliding window over the seven-way alignment. Lines represent 500 kb window size (step size 100 kb) sliding along the chromosome. The divergences are shown for the mat chromosome (LGI, left) and one of the autosomes (LGII, right). A: Intra-lineage comparisons (mat A vs mat a) of the three investigated lineages of N. tetrasperma: L1, L4 and L9. B: Comparisons of genomes from different lineages of N. tetrasperma (inter-lineage comparisons, dashed lines), and of N. tetrasperma and N. crassa (inter-species comparisons, solid lines). Note that the mat A – mat A inter-lineage comparisons (L1A–L4A, L1A–L9A and L4A–L9A) are plotted with blue lines. C: GC content in N. crassa (black line) and N. tetrasperma L1 (red line). Window size is 100 kb, step size 20 kb. Black bars indicate gene density in L1A.
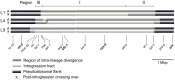
Regions of elevated intra-lineage divergence and introgression are defined based on genome divergence estimates. Genes positioned below the chromosomes were used for the phylogenetic and Bayesian concordance analyses. In bold are genes for which representatives of all known heterothallic species and subgroups of Neurospora were included in the phylogenetic analyses. The figure is drawn to scale.
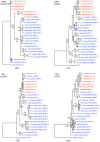
Each tree includes representatives of three N. tetrasperma lineages (red), and ten heterothallic Neurospora species (blue). Neurospora discreta was used as outgroup in all analyses. Chromosomal location (linkage group, right or left flank) is given within parenthesis by each locus name. The topologies shown are from the Maximum likelihood phylogenetic analyses, with the bootstrap supports for the analysis shown below the branches and the posterior probabilities from the Bayesian phylogenetic analysis shown above the branches.
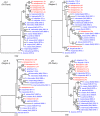
The genes nit-2 and phr are located in the left and right pseudoautosomal (PA) flank, respectively. The genes eth-1 and cys-9 are located in the central region of the chromosome, for which the mat a chromosomes of all lineages of N. tetrasperma (L1a, L4a and L9a) show indications of introgression. Each genealogy includes representatives of the three investigated N. tetrasperma lineages (red), and ten heterothallic Neurospora species (blue). Neurospora discreta was used as outgroup in all analyses. The topologies shown are from the Maximum likelihood phylogenetic analysis with the bootstrap supports for the analysis shown below the branches and the posterior probabilities from the Bayesian phylogenetic analysis shown above the branches.
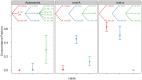
Bayesian concordance factors and 95% credibility intervals (error bars) estimated for 10 loci on the autosomes and 16 loci on the mat chromosomes. Concordance factors are given for the three hypotheses illustrated as clades in the plot, red: N. tetrasperma L9 sister species to N. crassa; blue: N. tetrasperma L4 sister species to N. hispaniola; green: N. tetrasperma monophyletic. The results displayed were calculated with the prior alpha set to 1.
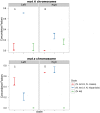
The concordance factors are plotted to the left and right of the crossover event described by Menkis et al. (2010) for both the mat A (A and B) and mat a (C and D) chromosomes. Concordance factors are given for the three hypotheses illustrated as clades in the plot, red: N. tetrasperma L9 sister species to N. crassa; blue: N. tetrasperma L4 sister species to N. hispaniola; green: N. tetrasperma monophyletic. The results displayed were calculated with the prior alpha set to 1.
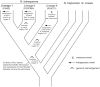
A putative scenario for the evolutionary processes leading to the results observed in this study. We speculate that a rearrangement on the mat A chromosome was associated with the evolution of pseudohomothallism in N. tetrasperma. During the subsequent divergence of the N. tetrasperma lineages, additional rearrangements, introgressions and a crossover took place.
Similar articles
-
Corcoran P, Anderson JL, Jacobson DJ, Sun Y, Ni P, Lascoux M, Johannesson H. Corcoran P, et al. Genome Res. 2016 Apr;26(4):486-98. doi: 10.1101/gr.197244.115. Epub 2016 Feb 18. Genome Res. 2016. PMID: 26893460 Free PMC article.
-
Menkis A, Jacobson DJ, Gustafsson T, Johannesson H. Menkis A, et al. PLoS Genet. 2008 Mar 14;4(3):e1000030. doi: 10.1371/journal.pgen.1000030. PLoS Genet. 2008. PMID: 18369449 Free PMC article.
-
Gladieux P, De Bellis F, Hann-Soden C, Svedberg J, Johannesson H, Taylor JW. Gladieux P, et al. Methods Mol Biol. 2020;2090:313-336. doi: 10.1007/978-1-0716-0199-0_13. Methods Mol Biol. 2020. PMID: 31975173 Review.
-
Whittle CA, Johannesson H. Whittle CA, et al. Bioessays. 2012 Nov;34(11):934-7. doi: 10.1002/bies.201200110. Epub 2012 Sep 12. Bioessays. 2012. PMID: 22968834 Review.
Cited by
-
Global population structure and adaptive evolution of aflatoxin-producing fungi.
Moore GG, Olarte RA, Horn BW, Elliott JL, Singh R, O'Neal CJ, Carbone I. Moore GG, et al. Ecol Evol. 2017 Sep 30;7(21):9179-9191. doi: 10.1002/ece3.3464. eCollection 2017 Nov. Ecol Evol. 2017. PMID: 29152206 Free PMC article.
-
Tempo of Degeneration Across Independently Evolved Nonrecombining Regions.
Carpentier F, Rodríguez de la Vega RC, Jay P, Duhamel M, Shykoff JA, Perlin MH, Wallen RM, Hood ME, Giraud T. Carpentier F, et al. Mol Biol Evol. 2022 Apr 11;39(4):msac060. doi: 10.1093/molbev/msac060. Mol Biol Evol. 2022. PMID: 35325190 Free PMC article.
-
Characterization of the reproductive mode and life cycle of the whitish truffle T. borchii.
Belfiori B, Riccioni C, Paolocci F, Rubini A. Belfiori B, et al. Mycorrhiza. 2016 Aug;26(6):515-27. doi: 10.1007/s00572-016-0689-0. Epub 2016 Mar 11. Mycorrhiza. 2016. PMID: 26968742
-
Maintenance of Species Boundaries Despite Ongoing Gene Flow in Ragworts.
Osborne OG, Chapman MA, Nevado B, Filatov DA. Osborne OG, et al. Genome Biol Evol. 2016 Apr 13;8(4):1038-47. doi: 10.1093/gbe/evw053. Genome Biol Evol. 2016. PMID: 26979797 Free PMC article.
-
A yeast living ancestor reveals the origin of genomic introgressions.
D'Angiolo M, De Chiara M, Yue JX, Irizar A, Stenberg S, Persson K, Llored A, Barré B, Schacherer J, Marangoni R, Gilson E, Warringer J, Liti G. D'Angiolo M, et al. Nature. 2020 Nov;587(7834):420-425. doi: 10.1038/s41586-020-2889-1. Epub 2020 Nov 11. Nature. 2020. PMID: 33177709
References
-
- Rieseberg LH, Ellstrand NC. Introgression and its consequences in plants. In Hybrid Zones and the Evolutionary Process. New York: Oxford University Press; 1993.
-
- Mallet J. Hybridization as an invasion of the genome. Trends Ecol Evol. 2005;20:229–237. - PubMed
Publication types
MeSH terms
LinkOut - more resources
Full Text Sources
Medical
Miscellaneous