The marine side of a terrestrial carnivore: intra-population variation in use of allochthonous resources by arctic foxes - PubMed
The marine side of a terrestrial carnivore: intra-population variation in use of allochthonous resources by arctic foxes
Arnaud Tarroux et al. PLoS One. 2012.
Abstract
Inter-individual variation in diet within generalist animal populations is thought to be a widespread phenomenon but its potential causes are poorly known. Inter-individual variation can be amplified by the availability and use of allochthonous resources, i.e., resources coming from spatially distinct ecosystems. Using a wild population of arctic fox as a study model, we tested hypotheses that could explain variation in both population and individual isotopic niches, used here as proxy for the trophic niche. The arctic fox is an opportunistic forager, dwelling in terrestrial and marine environments characterized by strong spatial (arctic-nesting birds) and temporal (cyclic lemmings) fluctuations in resource abundance. First, we tested the hypothesis that generalist foraging habits, in association with temporal variation in prey accessibility, should induce temporal changes in isotopic niche width and diet. Second, we investigated whether within-population variation in the isotopic niche could be explained by individual characteristics (sex and breeding status) and environmental factors (spatiotemporal variation in prey availability). We addressed these questions using isotopic analysis and bayesian mixing models in conjunction with linear mixed-effects models. We found that: i) arctic fox populations can simultaneously undergo short-term (i.e., within a few months) reduction in both isotopic niche width and inter-individual variability in isotopic ratios, ii) individual isotopic ratios were higher and more representative of a marine-based diet for non-breeding than breeding foxes early in spring, and iii) lemming population cycles did not appear to directly influence the diet of individual foxes after taking their breeding status into account. However, lemming abundance was correlated to proportion of breeding foxes, and could thus indirectly affect the diet at the population scale.
Conflict of interest statement
Competing Interests: The authors have declared that no competing interests exist.
Figures
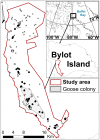
Locations of capture sites for breeding (•) and non-breeding (○) arctic foxes, monitored denning sites (X), and estimated average extent of the goose nesting colony during our study from 2003 to 2008 on Bylot Island (73°N, 80°W), Nunavut, Canada.
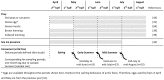
We used this phenological information to determine which prey was included in the mixing models for each fox dietary periods (Spring, Early-, and Mid-Summer). We assumed that δ13C and δ15N in whole blood represented the average diet during the previous month, hence the 1-month lag between dietary periods and their corresponding fox sampling periods (see methods and Fig. S1). Shaded areas are periods of availability (prey) or presence (sea ice).
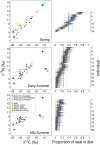
Left panel – Isotopic biplots of the isotopic signature (δ13C, δ15N) of arctic foxes and their potential prey sampled between 2003 and 2008. Dashed grey lines show the 95% CI dispersion ellipses based on standard deviation of foxes’ isotopic ratios for each period of the pup rearing season. Prey sample sizes are indicated in parentheses, unless identical to the previous period (see also Table S1). Spring: diet from mid-April to mid-May; Early-Summer: mid-May to mid-June; Mid-Summer: mid-June to mid-July (Fig. 2). Right panel – Corresponding SIAR output distributions of the relative proportion of marine sources (seal) in the reconstructed diet of each individual and by period. We show the mean (white dot) as well as the 50, 75, and 95% Credible Intervals (dark gray, light gray, and white boxes, respectively) of the SIAR posterior probability distributions. For each period, continuous and dotted lines (in blue) show the mean and 95% Credible Intervals at the population level, respectively.
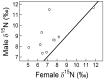
Dots above the line show pairs where the male had a higher δ15N than the female. Pearson’s r = 0.67, t = 2.57, df = 8, p = 0.033.
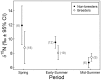
Average δ15N (‰ ±95% CI) of arctic foxes on Bylot Island, Nunavut, based on their breeding status and period of the pup rearing season. Spring: mid-April to mid-May; Early-Summer: mid-May to mid-June; Mid-Summer: mid-June to mid-July (Fig. 2). Numbers in parentheses indicate sample sizes and all data from years 2003–2008 were pooled, except those from four individuals whose breeding status could not be determined (Table 1).
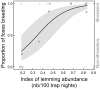
Proportion of breeding foxes captured annually as a function of the lemming snap-trapping index. The curve represents predictions from a generalized linear mixed-effects model fitted to the data (±1SE, shaded area around the curve). Small vertical bars (gray) represent the original data for breeders (top) or non-breeders (bottom) to which the model was fitted. The bars were jittered (randomly displaced over small distances on the X-axis) in order to better show data concentration. The open circles show the actual proportion of breeders for a given year/lemming index value. Total number of foxes captured each year is also available in Table 1. Data include only year 2004 to 2008 and lemming abundance data from 2004 to 2006 are drawn from Morrissette et al. , based on our long term monitoring of lemming abundance on Bylot Island (see methods for details).
Similar articles
-
Long-term responses of Icelandic Arctic foxes to changes in marine and terrestrial ecosystems.
Berthelot F, Unnsteinsdóttir ER, Carbonell Ellgutter JA, Ehrich D. Berthelot F, et al. PLoS One. 2023 Oct 4;18(10):e0282128. doi: 10.1371/journal.pone.0282128. eCollection 2023. PLoS One. 2023. PMID: 37792685 Free PMC article.
-
Giroux MA, Berteaux D, Lecomte N, Gauthier G, Szor G, Bêty J. Giroux MA, et al. J Anim Ecol. 2012 May;81(3):533-42. doi: 10.1111/j.1365-2656.2011.01944.x. Epub 2012 Jan 23. J Anim Ecol. 2012. PMID: 22268371
-
Ehrich D, Cerezo M, Rodnikova AY, Sokolova NA, Fuglei E, Shtro VG, Sokolov AA. Ehrich D, et al. BMC Ecol. 2017 Sep 16;17(1):32. doi: 10.1186/s12898-017-0142-z. BMC Ecol. 2017. PMID: 28915877 Free PMC article.
-
Climate change impacts on wildlife in a High Arctic archipelago - Svalbard, Norway.
Descamps S, Aars J, Fuglei E, Kovacs KM, Lydersen C, Pavlova O, Pedersen ÅØ, Ravolainen V, Strøm H. Descamps S, et al. Glob Chang Biol. 2017 Feb;23(2):490-502. doi: 10.1111/gcb.13381. Epub 2016 Jun 28. Glob Chang Biol. 2017. PMID: 27250039 Review.
-
Lemming and Vole Cycles: A New Intrinsic Model.
Levay EA, Nasser H, Zelko MD, Penman J, Johns TG. Levay EA, et al. Ecol Evol. 2024 Oct 21;14(10):e70440. doi: 10.1002/ece3.70440. eCollection 2024 Oct. Ecol Evol. 2024. PMID: 39440212 Free PMC article. Review.
Cited by
-
Yurkowski DJ, Ferguson S, Choy ES, Loseto LL, Brown TM, Muir DC, Semeniuk CA, Fisk AT. Yurkowski DJ, et al. Ecol Evol. 2016 Feb 14;6(6):1666-78. doi: 10.1002/ece3.1980. eCollection 2016 Mar. Ecol Evol. 2016. PMID: 26909143 Free PMC article.
-
Long-term responses of Icelandic Arctic foxes to changes in marine and terrestrial ecosystems.
Berthelot F, Unnsteinsdóttir ER, Carbonell Ellgutter JA, Ehrich D. Berthelot F, et al. PLoS One. 2023 Oct 4;18(10):e0282128. doi: 10.1371/journal.pone.0282128. eCollection 2023. PLoS One. 2023. PMID: 37792685 Free PMC article.
-
Highly overlapping winter diet in two sympatric lemming species revealed by DNA metabarcoding.
Soininen EM, Gauthier G, Bilodeau F, Berteaux D, Gielly L, Taberlet P, Gussarova G, Bellemain E, Hassel K, Stenøien HK, Epp L, Schrøder-Nielsen A, Brochmann C, Yoccoz NG. Soininen EM, et al. PLoS One. 2015 Jan 30;10(1):e0115335. doi: 10.1371/journal.pone.0115335. eCollection 2015. PLoS One. 2015. PMID: 25635852 Free PMC article.
-
Page HM, Schamel J, Emery KA, Schooler NK, Dugan JE, Guglielmino A, Schroeder DM, Palmstrom L, Hubbard DM, Miller RJ. Page HM, et al. PLoS One. 2021 Oct 28;16(10):e0258919. doi: 10.1371/journal.pone.0258919. eCollection 2021. PLoS One. 2021. PMID: 34710148 Free PMC article.
-
Cooper RJ, Krueger T, Hiscock KM, Rawlins BG. Cooper RJ, et al. Water Resour Res. 2014 Nov;50(11):9031-9047. doi: 10.1002/2014WR016194. Epub 2014 Nov 21. Water Resour Res. 2014. PMID: 26612962 Free PMC article.
References
-
- Estes JA, Riedman ML, Staedler MM, Tinker MT, Lyon BE (2003) Individual variation in prey selection by sea otters: patterns, causes and implications. Journal of Animal Ecology 72: 144–155.
-
- Araújo MS, Martins EG, Cruz LD, Fernandes FR, Linhares AX, et al. (2010) Nested diets: a novel pattern of individual-level resource use. Oikos 119: 81–88.
-
- Bolnick DI, Svanbäck R, Fordyce JA, Yang LH, Davis JM, et al. (2003) The ecology of individuals: incidence and implications of individual specialization. American Naturalist 161: 1–28. - PubMed
-
- Polis GA (1984) Age structure component of niche width and intraspecific resource partitioning: can age groups function as ecological species? American Naturalist 123: 541–564.
Publication types
MeSH terms
Grants and funding
This study was partly funded by an National Sciences & Engineering Research Council Northern Research Internship to A. Tarroux. This study was also supported by (alphabetical order): Canada Foundation for Innovation, Canada Research Chairs, Centre d'Études Nordiques, Environment Canada, Fonds Québécois de la Recherche sur la Nature et les Technologies, Indian and Northern Affairs Canada, Natural Sciences and Engineering Research Council of Canada (NSERC), Networks of Centres of Excellence of Canada ArcticNet, Nunavut Wildlife Management Board, Parks Canada, Polar Continental Shelf Program, Université du Québec à Rimouski (UQAR), and Université Laval. The funders had no role in the study design, data collection and analysis, decision to publish, or preparation of the manuscript.
LinkOut - more resources
Full Text Sources