Determining biophysical protein stability in lysates by a fast proteolysis assay, FASTpp - PubMed
Determining biophysical protein stability in lysates by a fast proteolysis assay, FASTpp
David P Minde et al. PLoS One. 2012.
Abstract
The biophysical stability is an important parameter for protein activity both in vivo and in vitro. Here we propose a method to analyse thermal melting of protein domains in lysates: Fast parallel proteolysis (FASTpp). Combining unfolding by a temperature gradient in a thermal cycler with simultaneous proteolytic cleavage of the unfolded state, we probed stability of single domains in lysates. We validated FASTpp on proteins from 10 kDa to 240 kDa and monitored stabilisation and coupled folding and binding upon interaction with small-molecule ligands. Within a total reaction time of approximately 1 min, we probed subtle stability differences of point mutations with high sensitivity and in agreement with data obtained by intrinsic protein fluorescence. We anticipate a wide range of applications of FASTpp in biomedicine and protein engineering as it requires only standard laboratory equipment.
Conflict of interest statement
Competing Interests: The authors have declared that no competing interests exist.
Figures
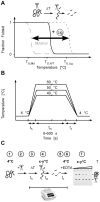
A, Protein stability can be probed by measuring the thermal unfolding transition in the presence of a protease. The folded state resists protease digestion while the unfolded state is readily digested on the same timescale. The thermal unfolding transition of a protein may be shifted to higher temperatures by addition of a ligand of the folded state. A shift to lower transition temperatures may occur upon destabilisation of the protein by, for instance, cancer mutations. B, Temperatures are controlled automatically using a standard gradient PCR setup. A mastermix of sample and protease is prepared on ice or in a cold room at 4°C and subsequently aliquoted to a PCR strip that is simultaneously heated up during the heating time th to a range of melting temperatures that are kept for a variable melting time tm. Subsequently simultaneous cooling (cooling time, tc ) brings all aliquots back to 4°C and the reaction is quenched by addition of EDTA. C, Scheme of all seven processing steps of the FASTpp assay. The representation of the termocycler indicates the automated steps of the FASTpp protocol, the gel indicates the final analysis by SDS-PAGE (T, temperature; ΔT, change of temperature; x–y°C, melting temperature gradient).
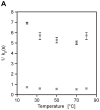
Thermal dependence of intrinsic rates of proteolysis of TL from 20°C to 80°C. After extensive thermal pre-equilibration, we determined kinetic traces of cleavage of an unstructured model substrate of TL, in triplicate for each temperature. The obtained rates were extrapolated based on empirically derived formulas to final concentrations of 0.1 g/L and on top 0.01 g/L of TL .
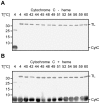
A, Thermal TL resistance of apo Cytochrome C (CytC). Only in absence of protease at 4°C, apo CytC was detected on the gel. Upon addition of TL, cleavage already occured on ice and no protease resistant protein was observed after additional incubation at higher temperatures. B, Thermal protease resistance of CytC in presence of the bound heme ligand. A strong band of CytC is visible in absence and presence of TL from temperatures from 4°C to 60°C.
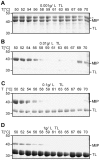
A, Thermal TL resistance of MBP using limiting TL concentration of 0.001 g/L. Over the entire temperature range from 50°C to 70°C, MBP remains intact. B, Thermal protease resistance of MBP using a TL concentration of 0.01 g/L. At this TL concentration, a clear thermal unfolding transition becomes apparent between 50°C and 60°C. Likely due to kinetic competition between irreversible aggregation and proteolytic cleavage of the unfolded state, some MBP is not digested at 69 and 70°C. C, Thermal protease resistance of MBP using limiting TL concentration of 0.1 g/L. A similar unfolding transition of MBP as in B is observed. D, Thermal protease resistance of MBP using limiting TL concentration of 1 g/L. A similar thermal unfolding transition of MBP is observed as in B.
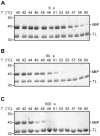
A, Thermal TL resistance of MBP using 6 s tm. MBP was increasingly cleaved from 40°C to 60°C. B, Thermal TL resistance of MBP using 60 s tm. MBP was increasingly accessible to digestion from 40°C to 53°C. Above 53°C, no MBP was detected. C, Thermal TL resistance of MBP using 600 s tm. MBP was increasingly accessible to digestion from 40°C to 49°C. Above 49°C temperature, no MBP was detected.
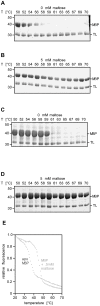
A, FASTpp of purified MBP. Unfolding was observed above 58°C. B, FASTpp of purified MBP plus 5 mM maltose. Unfolding was not observed up to 70°C. C, FASTpp proteolysis of MBP overexpression lysate. Unfolding was observed above 59°C. D, FASTpp of MBP overexpression lysate plus 5 mM maltose. Unfolding was not observed up to 70°C. E, Fluorescence melting curves of MBP. MBP melted in absence of a ligand between 30°C and 40°C. In presence of 5 mM maltose, unfolding was observed between 40°C and 50°C.
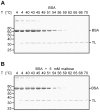
A, FASTpp of BSA in absence of maltose. BSA was completely digested at temperatures from 62°C to 70°C after a gradual unfolding transition over a range of temperatures from 51 to 59°C. B, FASTpp of BSA in presence of 5 mM maltose. BSA was completely digested at temperatures from 62°C to 70°C after a gradual unfolding transition over a range of temperatures from 51 to 59°C.
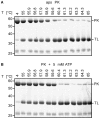
A, Pyruvat kinase (PK) FASTpp. PK was resistant from 4°C to 58°C. A gradual decrease in the band intensity at higher temperatures indicates unfolding. Over a broad range of even higher temperatures, a small fraction of protease-resistant species persists (that likely represent aggregates formed rapidly upon unfolding). B, FASTpp of PK in presence of 5 mM ATP. PK was resistant against TL digestion from 4°C to 59.6°C. Already at 60.4°C, nearly complete digestion was observed.
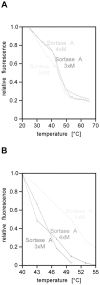
A, Intrinsic fluorescence temperature depence of three Sortase A variants. 3×M is triplemutant, 4×M is tetramutant, 5×M is pentamutant. B, FASTpp of the same three Sortase A variants as in A.
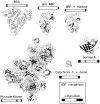
Representative snapshots from crystallographic studies on the used model proteins. BSA is α-helically folded (pdb identifier 1E7I), MBP has some β-sheets (pdb identifier 1JWY, 1ANF), PK contains more β-sheets (pdb identifier 1F3W), Sortase A mostly β-sheets (pdb identifier 1T2O) and folded Cytochrome C in presence of heme contains extended loops (pdb identifier 1AKK) , , , . The PONDR-FIT predictions are shown in black frames in a simplified view with black indicating a score for intrinsic disorder above 0.5 and background color scores from 0 to 0.5.
Similar articles
-
Investigating protein unfolding kinetics by pulse proteolysis.
Na YR, Park C. Na YR, et al. Protein Sci. 2009 Feb;18(2):268-76. doi: 10.1002/pro.29. Protein Sci. 2009. PMID: 19177560 Free PMC article.
-
Cozzi R, Zerbini F, Assfalg M, D'Onofrio M, Biagini M, Martinelli M, Nuccitelli A, Norais N, Telford JL, Maione D, Rinaudo CD. Cozzi R, et al. FASEB J. 2013 Aug;27(8):3144-54. doi: 10.1096/fj.13-227793. Epub 2013 Apr 30. FASEB J. 2013. PMID: 23631841
-
Sortase-mediated ligations for the site-specific modification of proteins.
Schmohl L, Schwarzer D. Schmohl L, et al. Curr Opin Chem Biol. 2014 Oct;22:122-8. doi: 10.1016/j.cbpa.2014.09.020. Epub 2014 Oct 6. Curr Opin Chem Biol. 2014. PMID: 25299574 Review.
-
Making and breaking peptide bonds: protein engineering using sortase.
Popp MW, Ploegh HL. Popp MW, et al. Angew Chem Int Ed Engl. 2011 May 23;50(22):5024-32. doi: 10.1002/anie.201008267. Epub 2011 Apr 27. Angew Chem Int Ed Engl. 2011. PMID: 21538739 Review.
-
A straight path to circular proteins.
Antos JM, Popp MW, Ernst R, Chew GL, Spooner E, Ploegh HL. Antos JM, et al. J Biol Chem. 2009 Jun 5;284(23):16028-36. doi: 10.1074/jbc.M901752200. Epub 2009 Apr 9. J Biol Chem. 2009. PMID: 19359246 Free PMC article.
Cited by
-
Minde DP, Radli M, Forneris F, Maurice MM, Rüdiger SG. Minde DP, et al. PLoS One. 2013 Oct 9;8(10):e77257. doi: 10.1371/journal.pone.0077257. eCollection 2013. PLoS One. 2013. PMID: 24130866 Free PMC article.
-
McAlary L, Nan JR, Shyu C, Sher M, Plotkin SS, Cashman NR. McAlary L, et al. Open Biol. 2024 Jun;14(6):230418. doi: 10.1098/rsob.230418. Epub 2024 Jun 5. Open Biol. 2024. PMID: 38835240 Free PMC article.
-
Insights into Protein Stability in Cell Lysate by 19 F NMR Spectroscopy.
Welte H, Kovermann M. Welte H, et al. Chembiochem. 2020 Dec 11;21(24):3575-3579. doi: 10.1002/cbic.202000413. Epub 2020 Sep 16. Chembiochem. 2020. PMID: 32786103 Free PMC article.
-
Designing disorder: Tales of the unexpected tails.
Minde DP, Halff EF, Tans S. Minde DP, et al. Intrinsically Disord Proteins. 2013 Jan 1;1(1):e26790. doi: 10.4161/idp.26790. eCollection 2013 Jan-Dec. Intrinsically Disord Proteins. 2013. PMID: 28516025 Free PMC article. Review.
-
High-Throughput Cellular Thermal Shift Assays in Research and Drug Discovery.
Henderson MJ, Holbert MA, Simeonov A, Kallal LA. Henderson MJ, et al. SLAS Discov. 2020 Feb;25(2):137-147. doi: 10.1177/2472555219877183. Epub 2019 Sep 30. SLAS Discov. 2020. PMID: 31566060 Free PMC article.
References
-
- Park C, Marqusee S (2005) Pulse proteolysis: A simple method for quantitative determination of protein stability and ligand binding. Nat Methods 2(3) 207–212: 10.1038/nmeth740. - PubMed
-
- Cabantous S, Pedelacq JD, Mark BL, Naranjo C, Terwilliger TC, et al. (2005) Recent advances in GFP folding reporter and split-GFP solubility reporter technologies. application to improving the folding and solubility of recalcitrant proteins from mycobacterium tuberculosis. J Struct Funct Genomics 6(2–3) 113–119: 10.1007/s10969–005-5247-5. - PubMed
-
- Desmazeaud MJ, Hermier JH (1971) Specificity of the nuclear protease produced by micrococcus caseolyticus. Eur J Biochem 19(1): 51–55. - PubMed
-
- Bark SJ, Muster N, Yates JR 3rd, Siuzdak G (2001) High-temperature protein mass mapping using a thermophilic protease. J Am Chem Soc 123(8): 1774–1775. - PubMed
Publication types
MeSH terms
Substances
Grants and funding
SGDR was supported by a Marie-Curie Excellence Grant of the European Union (MEXT-CT-2005-025651), a VIDI career development grant (700.55.421) by the Netherlands Organization for Scientific Research (NWO) and a High Potential Grant of Utrecht University. MMM was supported by the European Research Council (ERC-StG no.242958) and a High Potential Grant of Utrecht University. URLs of funders: NWO: http://www.nwo.nl/; EU: http://europa.eu/index_en.htm; Utrecht University: http://www.uu.nl/; ERC: http://erc.europa.eu/. The funders had no role in study design, data collection and analysis, decision to publish, or preparation of the manuscript.
LinkOut - more resources
Full Text Sources
Other Literature Sources