Fibroblast growth factor 10 gene regulation in the second heart field by Tbx1, Nkx2-5, and Islet1 reveals a genetic switch for down-regulation in the myocardium - PubMed
- ️Sun Jan 01 2012
Fibroblast growth factor 10 gene regulation in the second heart field by Tbx1, Nkx2-5, and Islet1 reveals a genetic switch for down-regulation in the myocardium
Yusuke Watanabe et al. Proc Natl Acad Sci U S A. 2012.
Abstract
During cardiogenesis, Fibroblast Growth Factor (Fgf10) is expressed in the anterior second heart field. Together with Fibroblast growth factor 8 (Fgf8), Fgf10 promotes the proliferation of these cardiac progenitor cells that form the arterial pole of the heart. We have identified a 1.7-kb region in the first intron of Fgf10 that is necessary and sufficient to direct transgene expression in this cardiac context. The 1.7-kb sequence is directly controlled by T-box transcription factor 1 (Tbx1) in anterior second heart field cells that contribute to the outflow tract. It also responds to both NK2 transcription factor related, locus 5 (Nkx2-5) and ISL1 transcription factor, LIM/homeodomain (Islet1), acting through overlapping sites. Mutation of these sites reduces transgene expression in the anterior second heart field where the Fgf10 regulatory element is activated by Islet1 via direct binding in vivo. Analysis of the response to Nkx2-5 loss- and Isl1 gain-of-function genetic backgrounds indicates that the observed up-regulation of its activity in Nkx2-5 mutant hearts, reflecting that of Fgf10, is due to the absence of Nkx2-5 repression and to up-regulation of Isl1, normally repressed in the myocardium by Nkx2-5. ChIP experiments show strong binding of Nkx2-5 in differentiated myocardium. Molecular and genetic analysis of the Fgf10 cardiac element therefore reveals how key cardiac transcription factors orchestrate gene expression in the anterior second heart field and how genes, such as Fgf10, normally expressed in the progenitor cell population, are repressed when these cells enter the heart and differentiate into myocardium. Our findings provide a paradigm for transcriptional mechanisms that underlie the changes in regulatory networks during the transition from progenitor state to that of the differentiated tissue.
Conflict of interest statement
The authors declare no conflict of interest.
Figures
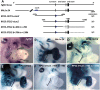
BAC transgenic analysis revealed that a +40 to +78-kb region of the Fgf10 locus is essential for transcriptional activity in the anterior SHF. (A) The Fgf10 locus, showing the site at −117 kb where two copies of the Mlc1v-nLacZ-24 transgene were inserted and the Fgf10-BAC constructs with the nLacZ reporter inserted at the translation start site of Fgf10. Blue arrowheads represent the nLacZ sequence, and dotted lines represent deleted regions 5′ (−) or 3′ (+). Distances are indicated in kilobases (kb) from the transcriptional start site of Fgf10. Exons are shown as black boxes. (B–G) Whole-mount views of the heart and adjacent structures of E9.5 mouse embryos. (B) Endogenous Fgf10 transcripts were detected by in situ hybridization in the pharyngeal mesoderm, including the anterior SHF (bracket) and weakly in the right ventricle (RV), but not in the outflow tract (OFT), both indicated by arrows. LV, left ventricle; A, right atrium, OV, otic vesicle. First and second pharyngeal arches are numbered. (C) X-gal staining of the Mlc1v-nLacZ-24 transgenic line recapitulated Fgf10 expression, with perduration of β-galactosidase in the outflow tract and right ventricle, resulting from the contribution of labeled cells from the anterior SHF (bracket). (D–G) X-gal staining of transgenic lines of Fgf10-BAC constructs directing the nLacZ reporter (A), with the anterior SHF indicated by a bracket. Numbers of embryos, at E9.5 with nLacZ expression in the anterior SHF (aSHF) compared with the total number of β-galactosidase–positive transgenic embryos is shown in the column on the right of A. A BAC containing −127 to +40 kb (RP24-157C5-nLacZ) did not show labeling in the anterior SHF (D), whereas a BAC containing −62 to +138 kb (R23-375J2-nLacZ) did (E). When the +40 to +78-kb region was deleted from the BAC containing +62 to +138 kb, the anterior SHF and outflow tract was not labeled [X-gal staining of the otic vesicle (OV) provided a positive control] (F), whereas when the +78 to +138-kb region was deleted from the BAC, β-galactosidase–positive cells were retained in the anterior SHF and outflow tract (G).
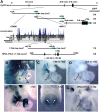
Transgenic analysis establishes that a 1.7-kb region at +45 kb in the Fgf10 gene is necessary and sufficient for expression in the anterior SHF. (A) Transgenes in which sequences within the first two introns and second and third exons (exons shown as black boxes), spanning +32 to +78 kb, of the Fgf10 gene were placed 5′ to the LacZ reporter (blue arrowhead), preceded by the hsp68 promoter (green box) are shown, together with the BAC RP23-375J2-nLacZ transgene (Fig. 1) in which the +44 to +46-kb regulatory region has been deleted (RP23-375J2Δ1.7-kb-nLacZ; dotted line). This covers the highly conserved 1.7-kb region shown in a cross-species sequence comparison, which was also placed in front of hsp-LacZ for transgenic analysis. Figures on the right indicate the number of transgenic embryos with expression in the anterior SHF (aSHF) compared with the total number of β-galactosidase–positive transgenic embryos at E9.5. (B–D) Examples of transgenic embryos stained with X-gal. At E9.5, labeling was seen in the anterior SHF (bracket), outflow tract (OFT, arrow), right ventricle (RV), and not in the left ventricle (LV) with +32 to +48 kb (1, 2, first and second pharyngeal arches) (B), was only detected in the arches with +48 to +63 kb (C), and with the +63 to +78-kb fragment expression was absent in the anterior SHF (bracket) (D). The 1.7-kb transgene directed expression in the anterior SHF (bracket), with β-galactosidase activity in its derivatives (outflow tract, right ventricle) in the heart at E9.5 (E) and in the SHF in the region lying medial to the cardiac crescent (arrows) at E7.5 (F). When the 1.7-kb sequence was deleted from the +62 to +138-kb BAC transgene (RP23-375J2Δ1.7-kb-nLacZ), no anterior SHF expression was seen (bracket), as shown at E9.5 (G).
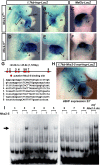
Nkx2-5 binds to the Fgf10 1.7-kb regulatory region and controls its transcriptional activity in the heart. (A–F) X-gal staining of E9.5 transgenic embryos, with the 1.7-kb-hsp-LacZ (A, B, D, and E) or Mef2c-aSHF-nLacZ (C and F) transgenes in a Nkx2-5 heterozygote (A–C) or homozygote mutant background (D–F). LacZ expression under the control of the 1.7-kb regulatory region was retained in the anterior SHF but ectopically induced in the single ventricle of Nkx2-5 mutants (D and E), whereas the Mef2c-aSHF enhancer did not change its expression pattern, which was limited to the remaining outflow region of the mutant heart (C and F). 1, 2, first and second pharyngeal arches; OFT, outflow tract; RV, right ventricle; LV, left ventricle; V, single ventricle in the mutant. (G) Localization of putative Nkx2-5 binding sites in the 1.7-kb regulatory region (+44/+48 kb) and the oligo DNA sequences (sites 1–8), which were used for EMSA. (H) The 1.7-kb regulatory region with mutations in all putative Nkx2.5 binding sites did not show ectopic β-galactosidase activity in the heart, and the activity decreased in the SHF (bracket). Two embryos in a total of seven β-galactosidase-positive embryos, at E9.5, had anterior (a) SHF expression. (I) EMSA with reticulocyte lysates containing Nkx2-5 protein (+) showed substantial Nkx2-5 binding (horizontal arrow) to sites 2 and 4 and binding to sites 3, 5, and 7 in the 1.7-kb region, compared with controls with lysate alone (−). In the two right-hand lanes an oligonucleotide with a consensus Nkx2-5 binding site from the atrial natriuretic factor (Anf/Nppa) gene (39) was used as a positive control.
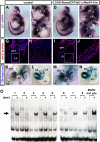
Islet1 directly activates the Fgf10 1.7-kb regulatory region in the heart. (A–G) In situ hybridization on E9.5 embryos showing Isl1 transcripts in control hearts (A–C) and transgenic hearts, where Isl1 was ectopically expressed (D–F). In control embryos (A), with enlargement of left lateral (B) and right lateral (C) views, Isl1 was expressed in the SHF (bracket, B and C) but not in the right ventricle (RV), left ventricle (LV), or atria. LA, left atria; OFT, outflow tract; 1, 2, first and second pharyngeal arches. The transgenic mouse that conditionally overexpresses Isl1 (CAGfloxedCAT-Isl1pA) was crossed with a Mesp1Cre/+ line to induce Islet1 expression throughout the heart (D–F), presented as in A–C. (G–J) Immunohistochemistry for Islet1 protein on sections of control hearts (G and H) and transgenic hearts (I and J). Islet1 protein was not detected in the left ventricle of control embryos at E9.5 (G and H), whereas it was present in the transgenic heart (red labeling in I and J). H and J are enlargements corresponding to the boxed area in G and I, respectively. (K–N) X-gal staining of control 1.7-kb-hsp-nLacZ transgenic embryos at E9.5 (K and L) and of these transgenic embryos on the CAG-floxedCAT-Isl1pA; Mesp1Cre/+ genetic background, where Islet1 was ectopically expressed in the heart. The outflow tract and right ventricle were β-galactosidase positive in the control heart (K and L), whereas the left ventricle and atria also showed X-gal labeling in the Islet1-positive hearts (M and N). K and M are lateral views of embryos in the cardiac region; L and N show frontal views of isolated hearts. (O) EMSAs with reticulocyte lysates with (+) or without (−) Islet1, with the oligonucleotides containing putative Nkx2-5 binding sites used in Fig. 3G, showed strong Islet1 binding to sites 1, 3, 4, 5, 7, and 8. The sequence in the Mef2c-aSHF enhancer that contains a consensus Islet1 binding site (16) provided a positive control (right lanes).
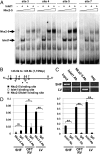
Nkx2-5 and Islet1 compete for homeodomain sites and bind to the Fgf10 regulatory region in vivo. (A) Competitive EMSA. When Nkx2-5 is added to the oligos 3, 4, 5, and 7 (Fig. 3G) in addition to Islet1, Islet1 binding was weakened, depending on Nkx2-5 dose. (B) Localization of Nkx2-5 and Islet1 binding sites. Positions of primers used for quantitative PCR (qPCR) and real-time qPCR are indicated by arrows and arrowheads, respectively. (C and D) ChIP analysis with E9.5 embryo extracts from the SHF, outflow tract (OFT), and right ventricle (RV) or left ventricle (LV), with Islet1 or Nkx2-5 antibodies. A representative result of qPCR with 5′ and 3′ primers for the 1.7-kb sequence is shown in C. Strong Nkx2-5 binding to the 1.7-kb regulatory region of Fgf10 in the left ventricle, but not in the SHF, was observed, whereas Islet1 was bound to the 1.7-kb sequence both in the SHF and left ventricle. ChIP results are summarized in D. Histograms show significant binding of Islet1 in all extracts and of Nkx2-5 in outflow tract/right ventricle and left ventricle extracts. *P < 0.05; **P < 0.01. Comparison of values on the y axis between extracts or antibodies is not valid because of limiting quantities of material for the former and differences in antibody performance for the latter.
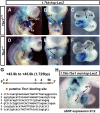
Tbx1 regulates the Fgf10 1.7-kb regulatory region in the anterior SHF. (A–F) X-gal staining of 1.7-kb-hsp-LacZ transgenic embryos at E9.5 on control (Tbx1+/+) (A–C) or Tbx1 mutant (Tbx1−/−) backgrounds (D–F). In control embryos, β-galactosidase–positive cells were present in the anterior SHF (bracket in A, white arrowheads in B), outflow tract (OFT), and right ventricle (RV) (A–C), whereas labeling in the anterior SHF and outflow tract was strongly reduced in Tbx1 mutant embryos (D–F). A and D are lateral views of the embryo; B and E are ventral views with the heart tube removed to show the SHF in the dorsal pericardial wall; and C and D show frontal views of isolated hearts. (G) There are six putative Tbx binding sites in the 1.7-kb sequence. (H) When these binding sites were mutated in the 1.7-kb-hsp-LacZ transgene, β-galactosidase–positive cells were lost in the anterior SHF (bracket) and outflow tract at E9.5. Some anterior SHF (aSHF) expression was observed in 3 of 12 transgenic embryos, with X-gal staining in the right ventricle.
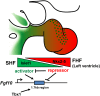
Model of Fgf10 regulation by Nkx2-5 and Islet1 in the heart. Nkx2-5 is expressed both in the progenitor cells of the SHF, which give rise to the arterial pole of the heart, and in the myocardium of the left ventricle, derived from the first heart field (FHF) (red) . The expression level of Nkx2-5 is higher in the myocardium. Islet1, on the other hand, is strongly expressed in the SHF. It is also expressed at a low level in the FHF, and it continues to be bound to chromatin in at least some cells in the myocardium, including in the left ventricle (green). The two factors bind homeodomain sites on the Fgf10 regulatory region, with overlapping specificities. Islet1 principally activates, and Nkx2-5 represses, transcription. Furthermore, Nkx2-5 negatively regulates Isl1 expression. This balance of Nkx2-5 and Islet1 determines the expression pattern of Fgf10, which is directly repressed by Nkx2-5 and requires Islet1 for activation. Activation of the 1.7-kb sequence also depends on T-box sites. In the SHF, Tbx1 is the principal activator, and we speculate that other Tbx factors, such as Tbx5, can play this role in the heart when Nkx2-5 repression is lifted.
Comment in
-
To activate or not to activate: the existential dilemma of an enhancer.
Boogerd CJ, Evans SM. Boogerd CJ, et al. Circ Res. 2013 Mar 29;112(7):985-7. doi: 10.1161/CIRCRESAHA.113.301133. Circ Res. 2013. PMID: 23538274 Free PMC article.
Similar articles
-
ISL1 directly regulates FGF10 transcription during human cardiac outflow formation.
Golzio C, Havis E, Daubas P, Nuel G, Babarit C, Munnich A, Vekemans M, Zaffran S, Lyonnet S, Etchevers HC. Golzio C, et al. PLoS One. 2012;7(1):e30677. doi: 10.1371/journal.pone.0030677. Epub 2012 Jan 27. PLoS One. 2012. PMID: 22303449 Free PMC article.
-
Kelly RG, Papaioannou VE. Kelly RG, et al. Dev Dyn. 2007 Mar;236(3):821-8. doi: 10.1002/dvdy.21063. Dev Dyn. 2007. PMID: 17238155
-
Tbx1 has a dual role in the morphogenesis of the cardiac outflow tract.
Xu H, Morishima M, Wylie JN, Schwartz RJ, Bruneau BG, Lindsay EA, Baldini A. Xu H, et al. Development. 2004 Jul;131(13):3217-27. doi: 10.1242/dev.01174. Epub 2004 Jun 2. Development. 2004. PMID: 15175244
-
Islet1-expressing cardiac progenitor cells: a comparison across species.
Pandur P, Sirbu IO, Kühl SJ, Philipp M, Kühl M. Pandur P, et al. Dev Genes Evol. 2013 Mar;223(1-2):117-29. doi: 10.1007/s00427-012-0400-1. Epub 2012 Apr 24. Dev Genes Evol. 2013. PMID: 22526874 Free PMC article. Review.
-
Cardiac transcription factor Csx/Nkx2-5: Its role in cardiac development and diseases.
Akazawa H, Komuro I. Akazawa H, et al. Pharmacol Ther. 2005 Aug;107(2):252-68. doi: 10.1016/j.pharmthera.2005.03.005. Pharmacol Ther. 2005. PMID: 15925411 Review.
Cited by
-
Location, Location, Location: Signals in Muscle Specification.
Chang CN, Kioussi C. Chang CN, et al. J Dev Biol. 2018 May 18;6(2):11. doi: 10.3390/jdb6020011. J Dev Biol. 2018. PMID: 29783715 Free PMC article. Review.
-
Control of cardiomyocyte differentiation timing by intercellular signaling pathways.
Rowton M, Guzzetta A, Rydeen AB, Moskowitz IP. Rowton M, et al. Semin Cell Dev Biol. 2021 Oct;118:94-106. doi: 10.1016/j.semcdb.2021.06.002. Epub 2021 Jun 16. Semin Cell Dev Biol. 2021. PMID: 34144893 Free PMC article. Review.
-
Disruption of spatiotemporal hypoxic signaling causes congenital heart disease in mice.
Yuan X, Qi H, Li X, Wu F, Fang J, Bober E, Dobreva G, Zhou Y, Braun T. Yuan X, et al. J Clin Invest. 2017 Jun 1;127(6):2235-2248. doi: 10.1172/JCI88725. Epub 2017 Apr 24. J Clin Invest. 2017. PMID: 28436940 Free PMC article.
-
Precardiac organoids form two heart fields via Bmp/Wnt signaling.
Andersen P, Tampakakis E, Jimenez DV, Kannan S, Miyamoto M, Shin HK, Saberi A, Murphy S, Sulistio E, Chelko SP, Kwon C. Andersen P, et al. Nat Commun. 2018 Aug 7;9(1):3140. doi: 10.1038/s41467-018-05604-8. Nat Commun. 2018. PMID: 30087351 Free PMC article.
-
In Vitro Generation of Heart Field-specific Cardiac Progenitor Cells.
Tampakakis E, Miyamoto M, Kwon C. Tampakakis E, et al. J Vis Exp. 2019 Jul 3;(149):10.3791/59826. doi: 10.3791/59826. J Vis Exp. 2019. PMID: 31329174 Free PMC article.
References
-
- Kelly RG, Brown NA, Buckingham ME. The arterial pole of the mouse heart forms from Fgf10-expressing cells in pharyngeal mesoderm. Dev Cell. 2001;1(3):435–440. - PubMed
-
- Zaffran S, Kelly RG, Meilhac SM, Buckingham ME, Brown NA. Right ventricular myocardium derives from the anterior heart field. Circ Res. 2004;95(3):261–268. - PubMed
-
- Galli D, et al. Atrial myocardium derives from the posterior region of the second heart field, which acquires left-right identity as Pitx2c is expressed. Development. 2008;135(6):1157–1167. - PubMed
-
- Meilhac SM, Esner M, Kelly RG, Nicolas JF, Buckingham ME. The clonal origin of myocardial cells in different regions of the embryonic mouse heart. Dev Cell. 2004;6(5):685–698. - PubMed
-
- Buckingham M, Meilhac S, Zaffran S. Building the mammalian heart from two sources of myocardial cells. Nat Rev Genet. 2005;6(11):826–835. - PubMed
Publication types
MeSH terms
Substances
LinkOut - more resources
Full Text Sources
Other Literature Sources
Molecular Biology Databases