Shikonin directly targets mitochondria and causes mitochondrial dysfunction in cancer cells - PubMed
Shikonin directly targets mitochondria and causes mitochondrial dysfunction in cancer cells
Benjamin Wiench et al. Evid Based Complement Alternat Med. 2012.
Abstract
Chemotherapy is a mainstay of cancer treatment. Due to increased drug resistance and the severe side effects of currently used therapeutics, new candidate compounds are required for improvement of therapy success. Shikonin, a natural naphthoquinone, was used in traditional Chinese medicine for the treatment of different inflammatory diseases and recent studies revealed the anticancer activities of shikonin. We found that shikonin has strong cytotoxic effects on 15 cancer cell lines, including multidrug-resistant cell lines. Transcriptome-wide mRNA expression studies showed that shikonin induced genetic pathways regulating cell cycle, mitochondrial function, levels of reactive oxygen species, and cytoskeletal formation. Taking advantage of the inherent fluorescence of shikonin, we analyzed its uptake and distribution in live cells with high spatial and temporal resolution using flow cytometry and confocal microscopy. Shikonin was specifically accumulated in the mitochondria, and this accumulation was associated with a shikonin-dependent deregulation of cellular Ca(2+) and ROS levels. This deregulation led to a breakdown of the mitochondrial membrane potential, dysfunction of microtubules, cell-cycle arrest, and ultimately induction of apoptosis. Seeing as both the metabolism and the structure of mitochondria show marked differences between cancer cells and normal cells, shikonin is a promising candidate for the next generation of chemotherapy.
Figures
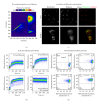
Shikonin accumulates in mitochondria and causes a breakdown of the mitochondrial membrane potential. (a) 2D excitation (200–600 nm) versus emission (300–700 nm) fluorescence spectrum of 50 μM shikonin in aqueous buffer. (b) Real-time kinetics and quantification of cellular shikonin uptake by flow cytometry. The inherent fluorescence of intracellular shikonin was measured at 640 nm excitation with a 730/45 nm bandpass filter. A dose-dependent increase of the cellular shikonin fluorescence was observed after treatment with increasing concentrations (0.15, 0.3 and 0.6 μM) of shikonin. After 20 min of incubation with 0.6 μM shikonin and subsequent washing of the cells, shikonin's fluorescence was still detectable in cells, indicating persistent intracellular accumulation of shikonin. (c) Shikonin localizes to mitochondria. U937 or SK-BR-3 cells were stained with MitoTracker Green and subsequently treated with 25 μM shikonin. Cells were then examined by confocal microscopy at an excitation wavelength of 488nm and 561 nm and emission at 500–549 nm and 680–780 nm for MitoTracker Green and shikonin, respectively. (d) Breakdown of the mitochondrial membrane potential. U937 cells were stained with JC-1, which has a strong red fluorescence in healthy mitochondria. Shikonin induced a dose-dependent decrease of the red JC-1 fluorescence after 6 h of treatment with increasing concentrations of shikonin (0.3, 0.6, and 1.2 μM), indicating a breakdown of the mitochondrial membrane potential.
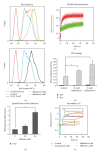
Induction of ROS, oxidative DNA damage, and elevated intracellular Ca2+ levels by shikonin. (a) Flow cytometric analysis of ROS levels after treatment with different concentrations of shikonin for 1 h or 50 μM H2O2 for 15 min in living U937 cells. Cells were stained with H2DCFH-DA and measured at 488 nm excitation and detected using a 530/30 nm bandpass filter. (b) Statistical quantification of ROS induction after shikonin treatment in U937 cells. Data points represent mean (fold change) ± SEM of at least three independent experiments. (c) ROS induction kinetics in live cells. U937 cells were stained with H2DCFH-DA and ROS induction was measured by flow cytometry. After 2 min, shikonin was added to the cells and measurement was continued for 1 h. Shikonin was excited at 640 nm and detected with a 730/45 nm bandpass filter. DCF was excited with a 488 nm laser and detected using a 530/30 nm bandpass filter. (d) Induction of DNA damage by shikonin measured using alkaline elution technique. Columns indicate the number of DNA single-strand breaks (SSB) and of Fpg-sensitive modifications (oxidative DNA damage) after shikonin treatment. Data points represent mean ± SEM of at least three independent experiments. (e) Real-time kinetics of intracellular Ca2+ levels after treatment with different concentrations of shikonin or ionomycin in U937 cells. Cells were stained with indo-1 and [Ca2+]i was measured by flow cytometry. After 2 min, shikonin was added to the cells and measurement was continued for 1 h. Indo-1 was excited with a 355 nm laser and the ratio of the signals detected using a 405/20 nm filter and a 530/30 filter (405/20 nm/530/30 nm) was used as an index for intracellular calcium concentration (*significant difference according to Student's t-test, P < 0.05).
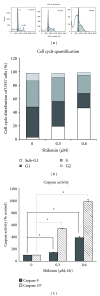
Shikonin induces cell cycle arrest and apoptosis in U937 cells. (a) Typical DNA content histograms of U937 cells treated with increasing concentrations of shikonin for 24 h. (b) Statistical analysis of cell cycle distribution of U937 cells after treatment with different concentrations of shikonin for 24 h. Data points are means of at least three independent experiments. (c) Enzymatic activity of caspase 3/7 and caspase 9 after 6 h of shikonin treatment in U937 cells. The caspase activity (mean ± SD of at least three experiments) is expressed as percentage relative to the untreated control (*significant difference according to Student's t-test, P < 0.05).
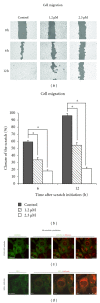
Shikonin inhibits cancer cell migration and affects microtubule structure and dynamics. (a) Typical pictures at 0, 6, and 12 h of scratch migration assays using SK-BR-3 cells treated with different effective, but in this time-frame subtoxic, concentrations of shikonin. (b) Statistical quantification of the scratch migration assay. Data points represent the mean ± SEM of at least three independent experiments. (c) Live imaging of U2OS-GFP-αTubulin cells stably transfected with a GFP fusion construct of α-tubulin and treated with 25 μM shikonin. With increasing cellular concentrations of shikonin, the number of distinct tubulin filaments decreased and the tubulin staining became progressively diffuse. (d) Live imaging of RPE-1-GFP-EB3 cells stably expressing GFP-EB3 and treated with 25 μM shikonin. Shikonin caused a slowdown and finally a complete disappearance of EB3 particles within 3min after application, indicating disrupted microtubule formation (*significant difference according to Student's t-test, P < 0.05).
Similar articles
-
Novel multiple apoptotic mechanism of shikonin in human glioma cells.
Chen CH, Lin ML, Ong PL, Yang JT. Chen CH, et al. Ann Surg Oncol. 2012 Sep;19(9):3097-106. doi: 10.1245/s10434-012-2324-4. Epub 2012 Mar 24. Ann Surg Oncol. 2012. PMID: 22446899
-
Shahsavari Z, Karami-Tehrani F, Salami S, Ghasemzadeh M. Shahsavari Z, et al. Tumour Biol. 2016 Apr;37(4):4479-91. doi: 10.1007/s13277-015-4258-5. Epub 2015 Oct 26. Tumour Biol. 2016. PMID: 26496737
-
He G, He G, Zhou R, Pi Z, Zhu T, Jiang L, Xie Y. He G, et al. Biochem Biophys Res Commun. 2016 Jan 22;469(4):1075-82. doi: 10.1016/j.bbrc.2015.12.100. Epub 2015 Dec 29. Biochem Biophys Res Commun. 2016. PMID: 26740178
-
Pharmacological properties and derivatives of shikonin-A review in recent years.
Guo C, He J, Song X, Tan L, Wang M, Jiang P, Li Y, Cao Z, Peng C. Guo C, et al. Pharmacol Res. 2019 Nov;149:104463. doi: 10.1016/j.phrs.2019.104463. Epub 2019 Sep 22. Pharmacol Res. 2019. PMID: 31553936 Review.
-
Research progress in mechanism of anticancer action of shikonin targeting reactive oxygen species.
Qi K, Li J, Hu Y, Qiao Y, Mu Y. Qi K, et al. Front Pharmacol. 2024 Jul 11;15:1416781. doi: 10.3389/fphar.2024.1416781. eCollection 2024. Front Pharmacol. 2024. PMID: 39076592 Free PMC article. Review.
Cited by
-
The anti-leukemia activity and mechanisms of shikonin: a mini review.
Dong H, Chang CD, Gao F, Zhang N, Yan XJ, Wu X, Wang YH. Dong H, et al. Front Pharmacol. 2023 Nov 2;14:1271252. doi: 10.3389/fphar.2023.1271252. eCollection 2023. Front Pharmacol. 2023. PMID: 38026987 Free PMC article. Review.
-
Gurard-Levin ZA, Liu C, Jekle A, Jaisinghani R, Ren S, Vandyck K, Jochmans D, Leyssen P, Neyts J, Blatt LM, Beigelman L, Symons JA, Raboisson P, Scholle MD, Deval J. Gurard-Levin ZA, et al. Antiviral Res. 2020 Oct;182:104924. doi: 10.1016/j.antiviral.2020.104924. Epub 2020 Sep 5. Antiviral Res. 2020. PMID: 32896566 Free PMC article.
-
Matthaiou EI, Guo Y, Barar J, Sandaltzopoulos R, Kandalaft LE, Li C, Coukos G, Omidi Y. Matthaiou EI, et al. Bioimpacts. 2022;12(1):65-86. doi: 10.34172/bi.2021.23511. Epub 2021 Dec 19. Bioimpacts. 2022. PMID: 35087718 Free PMC article.
-
Guo W, Wang X, Sun C, Wang J, Wang T. Guo W, et al. Korean J Physiol Pharmacol. 2023 Jul 1;27(4):357-364. doi: 10.4196/kjpp.2023.27.4.357. Korean J Physiol Pharmacol. 2023. PMID: 37386833 Free PMC article.
-
Markowitsch SD, Vakhrusheva O, Schupp P, Akele Y, Kitanovic J, Slade KS, Efferth T, Thomas A, Tsaur I, Mager R, Haferkamp A, Juengel E. Markowitsch SD, et al. Cancers (Basel). 2022 Feb 22;14(5):1114. doi: 10.3390/cancers14051114. Cancers (Basel). 2022. PMID: 35267423 Free PMC article.
References
-
- Jemal A, Bray F, Center MM, Ferlay J, Ward E, Forman D. Global cancer statistics. CA: Cancer Journal for Clinicians. 2011;61(2):69–90. - PubMed
-
- Hanahan D, Weinberg RA. Hallmarks of cancer: the next generation. Cell. 2011;144(5):646–674. - PubMed
-
- Fulda S, Debatin KM. Extrinsic versus intrinsic apoptosis pathways in anticancer chemotherapy. Oncogene. 2006;25(34):4798–4811. - PubMed
-
- Li P, Nijhawan D, Budihardjo I, et al. Cytochrome c and dATP-dependent formation of Apaf-1/caspase-9 complex initiates an apoptotic protease cascade. Cell. 1997;91(4):479–489. - PubMed
-
- Fulda S, Galluzzi L, Kroemer G. Targeting mitochondria for cancer therapy. Nature Reviews Drug Discovery. 2010;9(6):447–464. - PubMed
LinkOut - more resources
Full Text Sources
Miscellaneous