The genome of the obligate intracellular parasite Trachipleistophora hominis: new insights into microsporidian genome dynamics and reductive evolution - PubMed
doi: 10.1371/journal.ppat.1002979. Epub 2012 Oct 25.
Tom A Williams, Sirintra Nakjang, Christophe J Noël, Daniel C Swan, Alina V Goldberg, Simon R Harris, Thomas Weinmaier, Stephanie Markert, Dörte Becher, Jörg Bernhardt, Tal Dagan, Christian Hacker, John M Lucocq, Thomas Schweder, Thomas Rattei, Neil Hall, Robert P Hirt, T Martin Embley
Affiliations
- PMID: 23133373
- PMCID: PMC3486916
- DOI: 10.1371/journal.ppat.1002979
The genome of the obligate intracellular parasite Trachipleistophora hominis: new insights into microsporidian genome dynamics and reductive evolution
Eva Heinz et al. PLoS Pathog. 2012.
Abstract
The dynamics of reductive genome evolution for eukaryotes living inside other eukaryotic cells are poorly understood compared to well-studied model systems involving obligate intracellular bacteria. Here we present 8.5 Mb of sequence from the genome of the microsporidian Trachipleistophora hominis, isolated from an HIV/AIDS patient, which is an outgroup to the smaller compacted-genome species that primarily inform ideas of evolutionary mode for these enormously successful obligate intracellular parasites. Our data provide detailed information on the gene content, genome architecture and intergenic regions of a larger microsporidian genome, while comparative analyses allowed us to infer genomic features and metabolism of the common ancestor of the species investigated. Gene length reduction and massive loss of metabolic capacity in the common ancestor was accompanied by the evolution of novel microsporidian-specific protein families, whose conservation among microsporidians, against a background of reductive evolution, suggests they may have important functions in their parasitic lifestyle. The ancestor had already lost many metabolic pathways but retained glycolysis and the pentose phosphate pathway to provide cytosolic ATP and reduced coenzymes, and it had a minimal mitochondrion (mitosome) making Fe-S clusters but not ATP. It possessed bacterial-like nucleotide transport proteins as a key innovation for stealing host-generated ATP, the machinery for RNAi, key elements of the early secretory pathway, canonical eukaryotic as well as microsporidian-specific regulatory elements, a diversity of repetitive and transposable elements, and relatively low average gene density. Microsporidian genome evolution thus appears to have proceeded in at least two major steps: an ancestral remodelling of the proteome upon transition to intracellular parasitism that involved reduction but also selective expansion, followed by a secondary compaction of genome architecture in some, but not all, lineages.
Conflict of interest statement
The authors have declared that no competing interests exist.
Figures
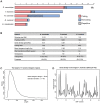
(A) Proportions of coding (red) and non-coding (blue) genomic DNA sequence for S. cerevisiae, T. hominis, N. ceranae, E. cuniculi and E. intestinalis. The repetitive content, which includes some coding as well as non-coding sequences, is indicated by the hatched overlay. The graph for E. cuniculi is based upon the 2.5 Mb of sequence that is available from Genbank and hence is less than the predicted genome size of 2.9 Mb. (B) Comparison of some general features of the genomes of S. cerevisiae, T. hominis and E. cuniculi. Numbers of transposons, transporters and proteases are those that could be identified based on similarity to characterized sequences in model organisms. (C) Density plot showing length variation of intergenic regions in the large T. hominis scaffolds. (D) Local length variation in intergenic regions over the largest (#00035, 311,951 bp) T. hominis scaffold.
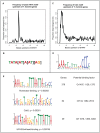
The intergenic regions upstream of T. hominis protein coding genes are enriched for both the canonical yeast-type TATA box (A, B) and a microsporidia-specific “CCC” motif (C, D). (E) The longer scaffolds of the T. hominis assembly also enabled us to robustly identify additional enriched motifs upstream from coding sequences (Figure S3, Table S4, S5). These included sequences with significant similarity to binding sites for Lim1-like homeobox-binding and fork head-containing transcription factors, as well as the cell cycle regulator Cdc5. The q-value for a match between a motif identified in T. hominis and the database consensus motif is a p-value that has been corrected for multiple testing by the False Discovery Rate method.
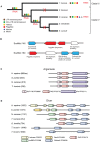
(A) Comparison of the TE content of T. hominis and N. ceranae suggests that the genome of the microsporidian ancestor contained several distinct families of transposons, which then appear to have been independently lost in some lineages (Table S6, Figures S4 to S7). Merlin transposons were only detected in the highly repetitive N. ceranae genome. (B) The genome of T. hominis contains PiggyBac DNA transposons which appear to have originated by LGT from a close relative of the ant Harpegnathos saltator (Figure S7), and thus independently from those detected in N. ceranae. The integration of the PiggyBac elements into the T. hominis genome was confirmed using PCR. (C, D) Domain conservation in the T. hominis and N. ceranae RNAi proteins Argonaute and Dicer. The domain architecture of Argonaute (C) and Dicer (D) were inferred using a pHMMER search of the respective sequences with default parameters. To identify more divergent domains in the Dicer homologues from parasites, the sequences of N. ceranae, T. hominis, T. brucei (1 and 2), and G. lamblia were investigated by searching the Pfam dataset from the conserved domain database at NCBI with an expected threshold of 100 . This identified the second N-terminal RNAse III domains of T. brucei Dicer 1 and Dicer 2, as well as an additional PAZ (Piwi, Argonaut and Zwille) domain for T. hominis. The Argonaute domain architecture is highly conserved among species, but Dicer is more variable and both microsporidian sequences as well as those of G. lamblia and T. brucei lack domains typically present in other organisms.
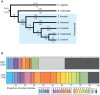
Homologous protein families were identified using MCL clustering of similar sequences, as determined by PHMMER searches (E<0.01). To prevent the clustering of otherwise unrelated proteins that share a common functional domain, we required that significantly similar sequence pairs align over at least 50% of their length. (A) Gain and loss events inferred using Dollo parsimony were mapped onto a cladogram derived from an 18S rRNA phylogeny . The numbers on the cladogram show the number of protein families lost (red) or gained (blue). (B) Functional classification of protein families gained or lost in the microsporidian ancestor using the COG functional categories, with the three broad divisions of informational genes, cellular processes and metabolism delineated. The microsporidian losses are distributed across COG categories, with a particular impact on cellular processes and metabolism, whereas most of the gains are of unknown function. Key: J, translation, ribosomal structure and biogenesis; A, RNA processing and modification; K, transcription; L, replication, recombination and repair; B, chromatin structure and dynamics; D, cell cycle control, cell division, chromosomal partitioning; Y, nuclear structure; V, defence mechanisms; T, signal transduction; M, cell wall/membrane/envelope biosynthesis; N, cell motility; Z, cytoskeleton; W, extracellular structures; U, intracellular trafficking, secretion, vesicular transport; O, posttranslational modification, protein turnover, chaperones; C, energy production and conversion; transport and metabolism of: G, carbohydrate; E, amino acids; F, nucleotides; H, coenzymes; I, lipids; P, inorganic ions; Q, secondary metabolites. R – general function prediction only; *, no whole-protein functional assignment, but contains a known functional region identified by HHsearch (Table S8); ?, no functional region identified using HHsearch.
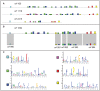
(A) Representative family members showing the characteristic pattern of LRR motifs. (B) Some shorter members of this family appear to be fragments derived from larger ORFs by pseudogenization, as indicated by patterns of sequence similarity and synteny. The height of the coloured boxes indicates the p-value of the motif hit assigned by the MEME suite . (C) The LRR motifs that form the building blocks of this protein family, which, at 117 members, is the largest identified in the T. hominis genome.
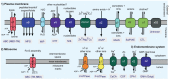
An overview of predicted T. hominis transporters with their possible locations in (1) the plasma membrane, (2) the mitosome, and (3) other endomembranes. The number of predicted proteins of each type is indicated in the icons; the predicted transport substrate (s) are also shown. Details of the predicted enzymes (EC numbers and descriptions) as well as the transporters (TC numbers and descriptions) are provided in the Tables S15 and S8, respectively.
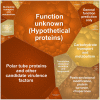
Voronoi treemap illustrating the major COG categories, complemented with an ad hoc category for putative T. hominis “virulence factors”, detected in the spore proteomics data. Each functional category and individual protein, for a total of 484 proteins (Table S19), are represented by delineated cells. The area of the different cells is proportional to the relative abundance of the proteins according to spectral counts. The locus tag and protein annotation for each individual protein are illustrated in the Voronoi treemap shown in Figure S16. Protein annotation, including predicted signal peptides and transmembrane domains, semi-quantitative spectral counts and presence of homologues of the 484 proteins in the other 5 microsporidians analysed, are provided in Table S19.
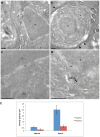
Cryosections of T. hominis labelled with anti-PGK-3 antiserum were sampled with micrographs taken at systematic uniform random (SUR) locations. The micrographs presented in the figure are representative of the quantitative data. (A) T. hominis in proliferative meront phase. White arrowheads indicate the plasma membrane of the parasite. (B) T. hominis early spore stage. Black arrowheads indicate the forming cell wall. (C) and (D) Enlarged image details from micrographs (A) and (B) respectively, demonstrating the difference in immunolabelling. In enlargement (D), PGK-3 labelling inside the cytosol can be seen (see black arrows). M = mitochondrion of the host rabbit kidney cell; Small arrows = mitosomes; PT = polar tube; N = nucleus; NE = nuclear envelope; bars = 200 nm. (E) Mean densities of PGK-3 gold labelling from three individual experiments. The area of compartments in T. hominis meronts and spore stages was estimated using point counting (see Materials and Methods) and counts of 10 nm gold made (n = 44 micrographs for spores, n = 51 micrographs for meronts; mean point counts were 92/experiment and mean gold counts 56/experiment). Error bars indicate the standard error of the mean.
Similar articles
-
Heinz E, Hacker C, Dean P, Mifsud J, Goldberg AV, Williams TA, Nakjang S, Gregory A, Hirt RP, Lucocq JM, Kunji ER, Embley TM. Heinz E, et al. PLoS Pathog. 2014 Dec 4;10(12):e1004547. doi: 10.1371/journal.ppat.1004547. eCollection 2014 Dec. PLoS Pathog. 2014. PMID: 25474405 Free PMC article.
-
Watson AK, Williams TA, Williams BA, Moore KA, Hirt RP, Embley TM. Watson AK, et al. BMC Genomics. 2015 Nov 21;16:983. doi: 10.1186/s12864-015-1989-z. BMC Genomics. 2015. PMID: 26589282 Free PMC article.
-
Reduction and expansion in microsporidian genome evolution: new insights from comparative genomics.
Nakjang S, Williams TA, Heinz E, Watson AK, Foster PG, Sendra KM, Heaps SE, Hirt RP, Martin Embley T. Nakjang S, et al. Genome Biol Evol. 2013;5(12):2285-303. doi: 10.1093/gbe/evt184. Genome Biol Evol. 2013. PMID: 24259309 Free PMC article.
-
The intriguing nature of microsporidian genomes.
Corradi N, Slamovits CH. Corradi N, et al. Brief Funct Genomics. 2011 May;10(3):115-24. doi: 10.1093/bfgp/elq032. Epub 2010 Dec 21. Brief Funct Genomics. 2011. PMID: 21177329 Review.
-
Energy metabolism and its evolution in Microsporidia and allied taxa.
Timofeev S, Tokarev Y, Dolgikh V. Timofeev S, et al. Parasitol Res. 2020 May;119(5):1433-1441. doi: 10.1007/s00436-020-06657-9. Epub 2020 Mar 21. Parasitol Res. 2020. PMID: 32200463 Review.
Cited by
-
From all to (nearly) none: Tracing adaptin evolution in Fungi.
Barlow LD, Dacks JB, Wideman JG. Barlow LD, et al. Cell Logist. 2014 Jan 1;4(1):e28114. doi: 10.4161/cl.28114. Epub 2014 Feb 21. Cell Logist. 2014. PMID: 24843829 Free PMC article.
-
Li Z, Wang Y, Wang L, Zhou Z. Li Z, et al. Parasit Vectors. 2018 Mar 6;11(1):147. doi: 10.1186/s13071-018-2755-2. Parasit Vectors. 2018. PMID: 29510742 Free PMC article.
-
Diversity and origins of anaerobic metabolism in mitochondria and related organelles.
Stairs CW, Leger MM, Roger AJ. Stairs CW, et al. Philos Trans R Soc Lond B Biol Sci. 2015 Sep 26;370(1678):20140326. doi: 10.1098/rstb.2014.0326. Philos Trans R Soc Lond B Biol Sci. 2015. PMID: 26323757 Free PMC article. Review.
-
Freibert SA, Goldberg AV, Hacker C, Molik S, Dean P, Williams TA, Nakjang S, Long S, Sendra K, Bill E, Heinz E, Hirt RP, Lucocq JM, Embley TM, Lill R. Freibert SA, et al. Nat Commun. 2017 Jan 4;8:13932. doi: 10.1038/ncomms13932. Nat Commun. 2017. PMID: 28051091 Free PMC article.
-
Genomic Survey of a Hyperparasitic Microsporidian Amphiamblys sp. (Metchnikovellidae).
Mikhailov KV, Simdyanov TG, Aleoshin VV. Mikhailov KV, et al. Genome Biol Evol. 2017 Mar 1;9(3):454-467. doi: 10.1093/gbe/evw235. Genome Biol Evol. 2017. PMID: 27694476 Free PMC article.
References
-
- Williams BA, Keeling PJ (2011) Microsporidia - highly reduced and derived relatives of Fungi. In: Poeggeler S, Woestemeyer J, editors. Evolution of Fungi and Fungal-like Organisms. Heidleberg: Springer. pp. 25–36.
-
- Wittner MW, Weiss L.M. (1999) The Microsporidia and Microsporidiosis. Washington D. C.: ASM Press. 553 p.
-
- Embley TM, Martin W (2006) Eukaryotic evolution, changes and challenges. Nature 440: 623–630. - PubMed
Publication types
MeSH terms
Substances
LinkOut - more resources
Full Text Sources
Other Literature Sources
Molecular Biology Databases
Miscellaneous