Metamorphic protein IscU alternates conformations in the course of its role as the scaffold protein for iron-sulfur cluster biosynthesis and delivery - PubMed
- ️Tue Jan 01 2013
Review
Metamorphic protein IscU alternates conformations in the course of its role as the scaffold protein for iron-sulfur cluster biosynthesis and delivery
John L Markley et al. FEBS Lett. 2013.
Abstract
IscU from Escherichia coli, the scaffold protein for iron-sulfur cluster biosynthesis and delivery, populates a complex energy landscape. IscU exists as two slowly interconverting species: one (S) is largely structured with all four peptidyl-prolyl bonds trans; the other (D) is partly disordered but contains an ordered domain that stabilizes two cis peptidyl-prolyl peptide bonds. At pH 8.0, the S-state is maximally populated at 25 °C, but its population decreases at higher or lower temperatures or at lower pH. The D-state binds preferentially to the cysteine desulfurase (IscS), which generates and transfers sulfur to IscU cysteine residues to form persulfides. The S-state is stabilized by Fe-S cluster binding and interacts preferentially with the DnaJ-type co-chaperone (HscB), which targets the holo-IscU:HscB complex to the DnaK-type chaperone (HscA) in its ATP-bound from. HscA is involved in delivery of Fe-S clusters to acceptor proteins by a mechanism dependent on ATP hydrolysis. Upon conversion of ATP to ADP, HscA binds the D-state of IscU ensuring release of the cluster and HscB. These findings have led to a more complete model for cluster biosynthesis and delivery.
Copyright © 2013 Federation of European Biochemical Societies. Published by Elsevier B.V. All rights reserved.
Figures
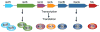
Representation of the Escherichia coli ISC operon and the proteins it encodes [7]. IscR is the homodimeric repressor protein [43] (34.6 kDa), which represses the operon when it contains a bound [2Fe–2S] cluster. IscS is the PLP-dependent cysteine desulfurase, which forms a homodimer (90.2 kDa) [44]. IscU is the scaffold protein for cluster assembly and transfer (13.8 kDa). The role of the IscA protein (11.6 kDa) remains controversial, although its X-ray crystal structure has been determined [45]. HscB is the DnaJ-type co-chaperone protein (20.1 kDa) that binds to holo-IscU and contains a J-domain with a conserved HPD amino acid motif that binds to the nucleotide-binding domain of HscA. HscA is the DnaK-type chaperone (65.7 kDa) that is involved in ATP-hydrolysis-driven cluster transfer. Fdx is ferredoxin (12.3 kDa) which binds a [2Fe–2S] cluster.
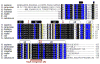
Alignment of the sequences of IscU proteins from major representative species. Note that the eukaryotic IscU proteins contain an N-terminal extension that targets the protein to mitochondria. Black indicates identically conserved residues, blue conserved residues, and yellow semi-conserved residues. The orange arrows point to the conserved cysteine residues that ligate the Fe-S cluster, and the pink arrow points to the conserved histidine residue that has been shown to be the fourth ligand to a [2Fe–2S] cluster in holo-IscU [19]. The IscU residues that form the HscA recognition site [37,46] are indicated by the horizontal red arrow.
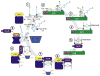
Working model for the role of IscU conformational states in the cycle of iron–sulfur cluster assembly and transfer [16,18,20]. (1) The free scaffold protein (IscU) exists in equilibrium between its two conformational states: a more structured state (S) with trans N13–P14 and P100–P101 peptide bonds and a partially disordered state (D) with cis N13–P14 and P100–P101 peptide bonds. (2) The PLP-dependent cysteine desulfurase (IscS), which catalyzes the conversion of cysteine to alanine to produce sulfane sulfur [47], preferentially binds to the D-state of IscU [18]. (3) Sulfur atoms are transferred from IscS to the cysteine side chains of IscU to form persulfides [9]; iron is then added. (4) The atoms rearrange to form a [2Fe–2S] cluster which stabilizes the S-state of IscU. (5) HscB, which preferentially binds the S-state of IscU, competes the holo-IscU off the cysteine desulfurase to form the HscB:holo-IscU complex. (6) The HPD motif in the J-domain of the co-chaperone (HscB), represented by the round knob, targets the HscB:holo-IscU complex to the nucleotide binding domain of HscA [38]. (7) An acceptor protein, represented by the blue trapezoid with four cysteinyl–SH groups approaches the complex. (8) Attack of cluster iron atoms by one or two (as shown) –SH groups liberates one or two (as shown) of the IscU cluster ligands, leading to a conformational change in HscB that activates the ATPase activity of HscA. (9) Cleavage of ATP bound to HscA to ADP leads to a conformational change [48,49] that opens up a binding site on the ligand binding domain of HscA that binds the D-state of IscU. Conversion of IscU from the S- to the D-state by binding to HscA(ADP), releases the cluster to the acceptor protein, and HscB. (9–1) Finally, exchange of bound ADP for ATP releases IscU, which then takes part in the next cycle.
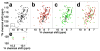
Evidence from 2D 1H–15N HSQC NMR spectra that E. coli IscU exists in solution as two slowly interconverting conformational states and that the equilibrium is shifted either by zinc ion binding or by the single-site mutation D39A. (a) Spectrum of E. coli IscU. Because IscU contains only a single Trp (W76), the presence of two cross-peaks in the boxed spectral region indicates the existence of two different conformations. (b) Spectrum of E. coli IscU in the presence of 3 mM ZnCl2 (red) overlaid with the spectrum of IscU (black). Zinc binding to IscU results in the disappearance of the minor Trp side chain peak as well as the cluster of broad peaks present in the central region of the spectrum of IscU. (c) Spectrum of E. coli IscU(D39A) (green) overlaid with the spectrum of IscU (black). As with zinc binding, the D39A substitution leads to the disappearance of the minor W76 side chain signal and the cluster of broad and overlapped peaks in the central region of the spectrum of IscU. (d) Spectrum of E. coli IscU in the presence of 3 mM ZnCl2 (red) overlaid with the spectrum of E. coli IscU(D39A) (green). The close correspondence between two spectra indicates that Zn-IscU and IscU(D39A) are structurally similar. (e) Two-dimensional 1H–15N Nz-exchange spectrum of IscU. Cross-peaks assigned to W76 (green) are connected by exchange cross-peaks (red). This result demonstrates that these two peaks originate not from two covalently distinct protein species but from two interchanging conformations. Figure adapted from [11].
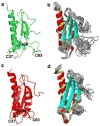
Three dimensional solution structures of the S-state of IscU and IscU(D39A) determined by NMR spectroscopy. (a) Structure of the S-state of IscU determined in the presence of ~20% D-state. The locations of the three cysteines that ligate the cluster are shown. (b) Family of models that represent the solution structure of IscU in the S-state. The N terminus is disordered as are the loops containing the cysteine cluster ligands. (c) Structure of IscU(D39A), a variant of IscU that is nearly 100% in the S-state. (d) Family of models that represent the solution structure of IscU(D39A). Note that the loops containing the cysteine cluster ligands are more ordered than in wild-type IscU. Figure adapted from [21].
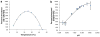
Temperature- and pH-dependence of the percent structured form of E. coli IscU, %S = ([S]/([S] + [D])) × 100. (a) Dependence of %S on temperature. The solution contained 1 mM IscU in a buffer consisting of 50 mM Tris–HCl (pH 8.0), 0.5 mM EDTA, 5 mM DTT, 150 mM NaCl, 10% D2O, 50 μM DSS, and 50 μM NaN3. (b) Dependence of %S on pH. The NMR sample contained 1 mM IscU, 0.5 mM EDTA, 5 mM DTT, 150 mM NaCl, 10% D2O, 50 μM DSS, and 50 μM NaN3. Figure adapted from [23].
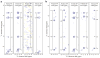
Evidence from 13C chemical shifts that all four peptidyl–prolyl peptide bonds in the S-state of IscU (stabilized by forming the Zn2+ complex at 25 °C) are trans, but that two peptidyl–prolyl peptide bonds (N13–P14 and P100–P101) are cis in the D-state (stabilized by raising the temperature to 45 °C). Shown are two-dimensional strips, taken at the chemical shifts of the prolyl 1Hα as indicated at the top of each strip, from 3D (H)CCH-TOCSY spectra of IscU acquired at 600 MHz (1H). The NMR sample contained 1 mM [U-13C, 15N-Pro]-IscU, 50 mM Tris–HCl (pH 8.0), 5 mM DTT, 150 mM NaCl, 50 μM DSS, and 50 μM NaN3 in 10% D2O. (a) Spectrum of the S-state of IscU stabilized as the Zn2+ complex at 25 °C. (b) Spectra of the D-state of IscU acquired at 45 °C. Figure from publication [16].
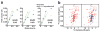
Evidence showing that IscS binds preferentially to and stabilizes the D-state of apo-IscU. (a) Volume of the S→D (left and center for Asn26 and Glu95, respectively) or D→S (Right for Asn26) Nz-exchange cross peak divided by the volume of the diagonal S or D peak, respectively, as a function of the mixing time in a two-dimensional NMR Nz-exchange experiment. Blue symbols are for IscU alone; green symbols are for IscU plus 0.1 equiv. of IscS. From the initial slopes, the S→D exchange rate increased from 0.77 s−1 for IscU alone to 1.1 s−1 for IscU in the presence of IscS; the D→S exchange rate decreased from 2.0 s−1 for IscU alone to 1.5 s−1 for IscU in the presence of IscS. The D⇆S equilibrium constant for apo-IscU from these measurements (0.24) is consistent with the relative populations of the S and D species determined from the 2D 1H–15N HSQC peak volumes under these conditions. (b) Two-dimensional 1H–15N NMR spectrum of apo-IscU labeled uniformly with nitrogen-15 (left; red) and the spectrum of the same sample mixed with a stoichiometric amount of unlabeled IscS (Right; blue) overlaid on the spectrum of IscU alone (right, red). Figure adapted from [18].
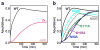
Time course of iron–sulfur cluster assembly as monitored by absorbance at 456 nm at room temperature in an anaerobic chamber (Coy Laboratory) filled with 90% N2 and 10% H2. The cluster assembly reaction contained 50 μM apo-IscU, 1 μM IscS, 250 μM ferrous ammonium sulfate, 0.1 M Tris–HCl (pH 7.5), 5 mM DTT. The reaction was initiated by adding L-cysteine to achieve a concentration of 250 μM. (a) Cluster assembly with wild-type IscU (black). Reaction in the absence of IscS (orange) showing that iron–sulfur cluster was not assembled in the absence of the cysteine desulfurase. Cluster assembly in the presence 250 μM Zn(Cl)2 (scarlet) showing that added Zn2+ inhibits cluster assembly. (b) Comparison of the time course of cluster assembly on IscU variants. Wild-type (WT) IscU assembled clusters more efficiently than the other five variants studied. The more structured variants (E111A, S107A, and N90A) assembled clusters following an initial lag and at a slower rate than WT. Like WT, the less structured variants (K89A and N90D) did not exhibit an initial lag, but they assembled clusters at rates intermediate between WT and the more structured variants. The decay of intensity after its highest point arises from the instability of the cluster once formed. Figure adapted from [18].
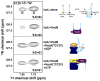
Conformational states of IscU under conditions mimicking various steps in the iron–sulfur cluster transfer mechanism. (Left) Backbone 1H–15N NMR peaks assigned to K128 in the D- and S-states of IscU. In each panel the peak from the D-state is on the left and that from the S-state is on the right. (Top panel) [U-15N]-IscU alone; (second panel) equimolar [U-15N]-IscU and HscB; (third panel) equimolar [U-15N]-IscU, HscB and HscA(T212 V) in the presence of excess ADP; (bottom panel) equimolar [U-15N]-IscU, HscB and HscA(T212 V) in the presence of excess ATP. (Right) To the right of each panel is a schematic figure representing the step being mimicked. Figure adapted from [20].
Similar articles
-
Bonomi F, Iametti S, Morleo A, Ta D, Vickery LE. Bonomi F, et al. Biochemistry. 2008 Dec 2;47(48):12795-801. doi: 10.1021/bi801565j. Biochemistry. 2008. PMID: 18986169
-
Kim JH, Tonelli M, Frederick RO, Chow DC, Markley JL. Kim JH, et al. J Biol Chem. 2012 Sep 7;287(37):31406-13. doi: 10.1074/jbc.M112.352617. Epub 2012 Jul 9. J Biol Chem. 2012. PMID: 22782893 Free PMC article.
-
Molecular chaperones HscA/Ssq1 and HscB/Jac1 and their roles in iron-sulfur protein maturation.
Vickery LE, Cupp-Vickery JR. Vickery LE, et al. Crit Rev Biochem Mol Biol. 2007 Mar-Apr;42(2):95-111. doi: 10.1080/10409230701322298. Crit Rev Biochem Mol Biol. 2007. PMID: 17453917 Review.
-
Kim JH, Tonelli M, Markley JL. Kim JH, et al. Proc Natl Acad Sci U S A. 2012 Jan 10;109(2):454-9. doi: 10.1073/pnas.1114372109. Epub 2011 Dec 27. Proc Natl Acad Sci U S A. 2012. PMID: 22203963 Free PMC article.
-
Kim JH, Bothe JR, Alderson TR, Markley JL. Kim JH, et al. Biochim Biophys Acta. 2015 Jun;1853(6):1416-28. doi: 10.1016/j.bbamcr.2014.11.020. Epub 2014 Nov 22. Biochim Biophys Acta. 2015. PMID: 25450980 Free PMC article. Review.
Cited by
-
Metamorphic proteins: the Janus proteins of structural biology.
Madhurima K, Nandi B, Sekhar A. Madhurima K, et al. Open Biol. 2021 Apr;11(4):210012. doi: 10.1098/rsob.210012. Epub 2021 Apr 21. Open Biol. 2021. PMID: 33878950 Free PMC article.
-
The role of zinc in the stability of the marginally stable IscU scaffold protein.
Iannuzzi C, Adrover M, Puglisi R, Yan R, Temussi PA, Pastore A. Iannuzzi C, et al. Protein Sci. 2014 Sep;23(9):1208-19. doi: 10.1002/pro.2501. Epub 2014 Jul 31. Protein Sci. 2014. PMID: 24917298 Free PMC article.
-
Morra S, Cordara A, Gilardi G, Valetti F. Morra S, et al. Protein Sci. 2015 Dec;24(12):2090-4. doi: 10.1002/pro.2805. Epub 2015 Sep 24. Protein Sci. 2015. PMID: 26362685 Free PMC article.
-
Altered levels of AtHSCB disrupts iron translocation from roots to shoots.
Leaden L, Pagani MA, Balparda M, Busi MV, Gomez-Casati DF. Leaden L, et al. Plant Mol Biol. 2016 Nov;92(4-5):613-628. doi: 10.1007/s11103-016-0537-9. Epub 2016 Sep 21. Plant Mol Biol. 2016. PMID: 27655366
-
Iametti S, Barbiroli A, Bonomi F. Iametti S, et al. J Biol Inorg Chem. 2015 Sep;20(6):1039-48. doi: 10.1007/s00775-015-1285-z. Epub 2015 Aug 6. J Biol Inorg Chem. 2015. PMID: 26246371
References
-
- Beinert H. Iron–sulfur proteins: ancient structures, still full of surprises. J Biol Inorg Chem. 2000;5:2–15. - PubMed
-
- Johnson DC, Dean DR, Smith AD, Johnson MK. Structure, function, and formation of biological iron–sulfur clusters. Annu Rev Biochem. 2005;74:247–281. - PubMed
-
- Lill R, Muhlenhoff U. Maturation of iron–sulfur proteins in eukaryotes: mechanisms, connected processes, and diseases. Annu Rev Biochem. 2008;77:669–700. - PubMed
-
- Sheftel A, Stehling O, Lill R. Iron–sulfur proteins in health and disease. Trends Endocrinol Metab. 2010;21:302–314. - PubMed
Publication types
MeSH terms
Substances
Grants and funding
LinkOut - more resources
Full Text Sources
Other Literature Sources
Molecular Biology Databases
Miscellaneous