Programmable plasmid interference by the CRISPR-Cas system in Thermococcus kodakarensis - PubMed
Programmable plasmid interference by the CRISPR-Cas system in Thermococcus kodakarensis
Joshua R Elmore et al. RNA Biol. 2013 May.
Abstract
CRISPR-Cas systems are RNA-guided immune systems that protect prokaryotes against viruses and other invaders. The CRISPR locus encodes crRNAs that recognize invading nucleic acid sequences and trigger silencing by the associated Cas proteins. There are multiple CRISPR-Cas systems with distinct compositions and mechanistic processes. Thermococcus kodakarensis (Tko) is a hyperthermophilic euryarchaeon that has both a Type I-A Csa and a Type I-B Cst CRISPR-Cas system. We have analyzed the expression and composition of crRNAs from the three CRISPRs in Tko by RNA deep sequencing and northern analysis. Our results indicate that crRNAs associated with these two CRISPR-Cas systems include an 8-nucleotide conserved sequence tag at the 5' end. We challenged Tko with plasmid invaders containing sequences targeted by endogenous crRNAs and observed active CRISPR-Cas-mediated silencing. Plasmid silencing was dependent on complementarity with a crRNA as well as on a sequence element found immediately adjacent to the crRNA recognition site in the target termed the PAM (protospacer adjacent motif). Silencing occurred independently of the orientation of the target sequence in the plasmid, and appears to occur at the DNA level, presumably via DNA degradation. In addition, we have directed silencing of an invader plasmid by genetically engineering the chromosomal CRISPR locus to express customized crRNAs directed against the plasmid. Our results support CRISPR engineering as a feasible approach to develop prokaryotic strains that are resistant to infection for use in industry.
Keywords: CRISPR; Cas; DNA; RNA; Thermococcus; archaea; hyperthermophile; immune; interference; silencing.
Figures
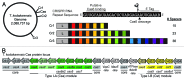
Figure 1. The CRISPR-Cas system of Thermococcus kodakarensis. (A) Relative locations of the CRISPRs (Cr1, Cr2, Cr3) and cas gene cluster (Cas) are indicated on the Tko genome. The consensus crRNA repeat sequence found in the three CRISPR loci is shown, and the proposed Cas6 binding and cleavage sites as well as the 8-nt 5′ tag sequence (5′AUUGAAAB 3′; where B = C, G, T) found on the mature crRNAs are highlighted. The relative size and spacer counts for each of the CRISPR loci are indicated and CRISPRs are arranged with leader regions (L) preceding repeat (black) and guide/spacer (colored) elements. (B) The genome organization and annotations of the predicted cas genes were adapted from the NCBI database (
http://www.ncbi.nlm.nih.gov/). Type I-A Csa, Type I-B Cst and core cas genes are indicated by green, yellow and gray, respectively with recent cas gene superfamily designations indicated below relevant csa and cst genes. Three non-cas genes are found within the Cas protein locus. TK0459 is annotated as an archaeal ATPase with a MarB-like DNA-binding domain. TK0456 and TK0457 appear to be a potential toxin/anti-toxin pair, annotated as a VapC-like (toxin) protein and a MazE-like (anti-toxin) protein.
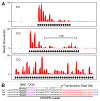
Figure 2. CRISPR RNAs are produced by all three CRISPRs in T. kodakarensis. (A) Small RNA sequencing reads mapped to the three CRISPR loci. CRISPRs are oriented with the leader on the left and the Y-axis represents thousands of reads. An X-axis scale of 1 kb is indicated and CRISPR repeats (black boxes) are indicated beneath the reads for each CRISPR locus. The RNAs transcribed from the leader region (red) are plotted above the X-axis while much rarer RNAs generated from the opposite strand (blue) are plotted below the X-axis. Images were generated using the UCSC archaeal genome browser. (B) Predicted promoter elements found in the 3′ end of the leader sequences. The sequences are aligned relative to the first leader-proximal repeat in each of the CRISPRs. Putative BRE and TATA box elements are indicated. Transcribed leader sequences (red) are indicated upstream of the first repeat (blue). Consensus BRE/TATA elements and predicted transcription start sites are shown above the sequence alignment.
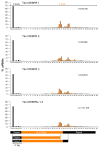
Figure 3. End analysis of sequenced crRNAs. The Y-axis represents the percentage of all crRNAs sequenced. The 5′ and 3′ ends of all crRNAs are mapped relative to the upstream repeat/guide junction for each of the three CRISPR loci as well as for all three. The 5′ ends (black bars) and 3′ ends (orange bars) of all crRNAs sequenced are indicated. Beneath the plot, the corresponding positions of a CRISPR repeat (black) and guide sequence (orange) are indicated. The model illustrates the most common Tko guide sequence length (38 nts). The two most common crRNA species (with 36 and 43 nts downstream of the 5′ repeat tag) are diagrammed below.
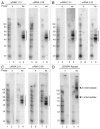
Figure 4. Northern analysis of crRNA expression. Blots were probed for two representative crRNAs from each of the three CRISPR loci (A–C) as well as the CRISPR repeat sequence (D). For each panel, both sense (s) and antisense (as) probes were tested. Odd numbered lanes contain the Decade Marker RNA, and even numbered lanes indicate lanes containing 10 µg of Tko total RNA. The crRNA species are indicated (brackets, A–C). In (D), RNAs corresponding in size to the predicted Cas6 cleavage products (1x: ~70 nt and 2x: ~140 nt) are indicated.
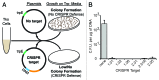
Figure 5. T. kodakarensis CRISPR-Cas systems silence plasmid DNA. (A) Graphic representation of the plasmid challenge assay. In the absence of CRISPR-Cas defense, the pLC64 plasmid, bearing a trpE marker gene cassette, is able to restore tryptophan prototrophy when transformed into Tko strain KUW1 (∆pyrF∆trpE). However, when derivatives of pLC64 bearing a CRISPR-Cas target sequence and a PAM are transformed, CRISPR-Cas silencing results in reduced or no colony formation on tryptophan-deficient medium. When silencing occurs by targeting DNA, the relative orientation of the target on the plasmid has no effect on silencing. (B) Plasmid challenge assay results. Colony formation per µg of plasmid DNA transformed is plotted on the Y-axis with error bars showing the standard error in five assay replicates. The target sequence of each plasmid is displayed on the X-axis. Nomenclature for the CRISPR targets is as follows: 2.01 indicates the protospacer target derived from the first leader proximal spacer of CRISPR locus 2, etc. The 2.01R target sequence is identical to the 2.01 target sequence, but its relative orientation on the plasmid is reversed. The assay was performed in two separate labs with a total of five replicates, using two different plasmid preparations.
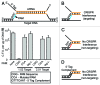
Figure 6. CRISPR-Cas plasmid interference is Protospacer Adjacent Motif (PAM)-dependent. (A) Diagram of crRNA base-pairing with targeted DNA molecule. The guide sequence of the crRNA (orange), derived from the spacer, is shown base pairing to its complement strand in the target (orange). Repeat sequences of the crRNA (black) and the PAM region (blue) are indicated (B–D). Diagrams for comparing three constructs with different protospacer flanking sequences used to test the mode of self vs. non-self determination in Tko CRISPR-Cas defense. (B) Targeting via detection of a PAM (tested with a predicted PAM sequence 5′-CGG-3′, blue). (C) Avoidance of CRISPR-Cas targeting via mutation of the PAM to a non-PAM sequence (tested with predicted non-PAM sequence 5′-CCA-3′, red). (D) Avoidance of CRISPR-Cas targeting via base-pairing between the 8 nt of the 5′-tag sequence and the sequence immediately downstream of the region complementary to the guide (tested with the 5′-tag complement sequence 5′-GTTTCAAT-3′, black). (E) Plasmid challenge assay results. Colony formation per µg of plasmid DNA transformed is plotted on the Y-axis with error bars displaying the standard error in six assay replicates, using two different plasmid preparations. The target sequence and the flanking region (PAM) of each plasmid are displayed on the X-axis.
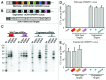
Figure 7. Engineered CRISPR1 locus guides CRISPR-Cas to target plasmid. Graphic representation of the 5′ ends of the wild-type CRISPR1 locus found in strains KOD1 and KUW1 (A) and the engineered CRISPR1 locus found in the strain aChiA, and the chiA∆4 gene (B). Engineered spacers and their corresponding targets within the chiA∆4 gene are indicated by light blue boxes. (C) Northern analysis of wild-type crRNA (1.01, 1.06) or engineered crRNA (A and E) expression. Lanes contain either Decade Marker RNA (M) or 10 µg of total RNA isolated from the wild-type CRISPR1 strains (KOD1 and KUW1) and engineered CRISPR1 strain (aChiA). Colored boxes above each blot indicate the crRNA guide sequence probed in each experiment. Plasmid challenge assay results using the KUW1 (WT CRISPR1) strain (D) or the aChiA (Engineered CRISPR1) strain (E). Colony formation per µg of plasmid DNA transformed is plotted on the Y-axis with error bars show the standard error in four replicate experiments performed by two separate labs. Wild-type CRISPR and engineered CRISPR plasmid targets are indicated on the X-axis, with colored boxes indicating the origin of the crRNA guiding the potential plasmid silencing. ChiA∆4 target plasmids contain the chiA∆4 expression cassette.
Similar articles
-
The RNA- and DNA-targeting CRISPR-Cas immune systems of Pyrococcus furiosus.
Terns RM, Terns MP. Terns RM, et al. Biochem Soc Trans. 2013 Dec;41(6):1416-21. doi: 10.1042/BST20130056. Biochem Soc Trans. 2013. PMID: 24256230 Free PMC article. Review.
-
Three CRISPR-Cas immune effector complexes coexist in Pyrococcus furiosus.
Majumdar S, Zhao P, Pfister NT, Compton M, Olson S, Glover CV 3rd, Wells L, Graveley BR, Terns RM, Terns MP. Majumdar S, et al. RNA. 2015 Jun;21(6):1147-58. doi: 10.1261/rna.049130.114. Epub 2015 Apr 22. RNA. 2015. PMID: 25904135 Free PMC article.
-
Maier LK, Stachler AE, Saunders SJ, Backofen R, Marchfelder A. Maier LK, et al. J Biol Chem. 2015 Feb 13;290(7):4192-201. doi: 10.1074/jbc.M114.617506. Epub 2014 Dec 15. J Biol Chem. 2015. PMID: 25512373 Free PMC article.
-
Maier LK, Lange SJ, Stoll B, Haas KA, Fischer S, Fischer E, Duchardt-Ferner E, Wöhnert J, Backofen R, Marchfelder A. Maier LK, et al. RNA Biol. 2013 May;10(5):865-74. doi: 10.4161/rna.24282. Epub 2013 Apr 17. RNA Biol. 2013. PMID: 23594992 Free PMC article.
-
Approaches to study CRISPR RNA biogenesis and the key players involved.
Behler J, Hess WR. Behler J, et al. Methods. 2020 Feb 1;172:12-26. doi: 10.1016/j.ymeth.2019.07.015. Epub 2019 Jul 17. Methods. 2020. PMID: 31325492 Review.
Cited by
-
The RNA- and DNA-targeting CRISPR-Cas immune systems of Pyrococcus furiosus.
Terns RM, Terns MP. Terns RM, et al. Biochem Soc Trans. 2013 Dec;41(6):1416-21. doi: 10.1042/BST20130056. Biochem Soc Trans. 2013. PMID: 24256230 Free PMC article. Review.
-
CRISPR/Cas9-mediated viral interference in plants.
Ali Z, Abulfaraj A, Idris A, Ali S, Tashkandi M, Mahfouz MM. Ali Z, et al. Genome Biol. 2015 Nov 11;16:238. doi: 10.1186/s13059-015-0799-6. Genome Biol. 2015. PMID: 26556628 Free PMC article.
-
Marchfelder A. Marchfelder A. RNA Biol. 2013 May;10(5):655-8. doi: 10.4161/rna.24687. RNA Biol. 2013. PMID: 23872677 Free PMC article. No abstract available.
-
Three CRISPR-Cas immune effector complexes coexist in Pyrococcus furiosus.
Majumdar S, Zhao P, Pfister NT, Compton M, Olson S, Glover CV 3rd, Wells L, Graveley BR, Terns RM, Terns MP. Majumdar S, et al. RNA. 2015 Jun;21(6):1147-58. doi: 10.1261/rna.049130.114. Epub 2015 Apr 22. RNA. 2015. PMID: 25904135 Free PMC article.
-
CRISPR-based technologies: prokaryotic defense weapons repurposed.
Terns RM, Terns MP. Terns RM, et al. Trends Genet. 2014 Mar;30(3):111-8. doi: 10.1016/j.tig.2014.01.003. Epub 2014 Feb 18. Trends Genet. 2014. PMID: 24555991 Free PMC article. Review.
References
Publication types
MeSH terms
Substances
Grants and funding
LinkOut - more resources
Full Text Sources
Other Literature Sources
Miscellaneous