RRM2 regulates Bcl-2 in head and neck and lung cancers: a potential target for cancer therapy - PubMed
- ️Tue Jan 01 2013
RRM2 regulates Bcl-2 in head and neck and lung cancers: a potential target for cancer therapy
Mohammad Aminur Rahman et al. Clin Cancer Res. 2013.
Abstract
Purpose: Ribonucleotide reductase subunit M2 (RRM2) plays an active role in tumor progression. Recently, we reported that depletion of RRM2 by systemic delivery of a nanoparticle carrying RRM2-specific siRNA suppresses head and neck tumor growth. The aim of this study is to clarify the underlying mechanism by which RRM2 depletion inhibits tumor growth.
Experimental design: siRNA-mediated gene silencing was carried out to downregulate RRM2. Immunoblotting, reverse-transcriptase PCR, confocal microscopy, tissue fractionation, gene overexpression and knockdown were employed to analyze critical apoptosis signaling. Conventional immunohistochemistry and quantum dot-based immunofluorescence were applied to detect RRM2 and Bcl2 expression and localization in tissue samples from patients and mice.
Results: Knockdown of RRM2 led to apoptosis through the intrinsic pathway in head and neck squamous cell carcinoma (HNSCC) and non-small cell lung cancer (NSCLC) cell lines. We showed that Bcl-2 is a key determinant controlling apoptosis, both in vitro and in vivo, and that RRM2 depletion significantly reduces Bcl-2 protein expression. We observed that RRM2 regulates Bcl-2 protein stability, with RRM2 suppression leading to increased Bcl-2 degradation, and identified their colocalization in HNSCC and NSCLC cells. In a total of 50 specimens each from patients with HNSCC and NSCLC, we identified the colocalization of Bcl-2 and RRM2 and found a significant positive correlation between their expression in HNSCC (R = 0.98; P < 0.0001) and NSCLC (R = 0.92; P < 0.0001) tumor tissues.
Conclusions: Our novel findings add to the knowledge of RRM2 in regulating expression of the antiapoptotic protein Bcl-2 and reveal a critical link between RRM2 and Bcl-2 in apoptosis signaling.
©2013 AACR.
Conflict of interest statement
Disclosure of Potential Conflicts of Interest
There is no potential conflict of interest to authors.
Figures
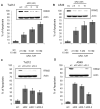
Knockdown of RRM2 induced apoptosis. Apoptosis was measured by annexin V-PE and 7-AAD staining in (A)Tu212 and (B) A459 cell lines 72h after siRNA transfection (error bars are mean ±SD from 3 independent experiments). Cell lysates were analyzed by Western blotting with the indicated antibodies after transfection (Inset). (C) Tu212 and (D) A549 cells were transfected with siC or different siRNAs against RRM2, siR2, siR2-1, siR2-2. After 72 h, apoptosis was measured by annexin V-PE and 7-AAD staining. Cell lysates were analyzed by Western blotting with the indicated antibodies after transfection (Inset).
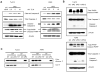
Knockdown of RRM2 induces apoptosis via the mitochondria-mediated intrinsic pathway. (A) Tu212 (left panel) and A549 (right panel) cells were transfected with siC or siR2. After 72 h, cell lysates were analyzed by Western blotting with the indicated antibodies. A single set of blots is pictured from three independent experiments. (B) Tu212 (upper panel) and A549 (lower panel) cells were transfected with siC or different siRNAs against RRM2, siR2, siR2-1, siR2-2. After 72 h, cell lysates were analyzed by Western blotting with the indicated antibodies. A single set of blots is pictured from three independent experiments. (C) Mitochondrial fraction (MF) and cytosolic fraction (CF) of Tu212 and A549 cells were isolated 72h after transfection with 5 nM siR2, 5 nM siC, or no treatment (NT). Western blotting was performed to detect Cytochrome C and Cox-4.
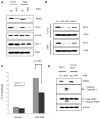
Apoptosis induction by knockdown of RRM2 is Bcl-2 dependent. (A) Cell lysates of Tu212 and A549 were collected 72h after transfection with 5 nM siC or siR2. Western blotting was performed to detect anti-apoptotic Bcl-2 family proteins. (B) Cell lysates of Tu212 and A549 were collected 72h after transfection with 5 nM siC or different siRNAs against RRM2, siR2, siR2-1, siR2-2. Western blotting was performed to detect Bcl-2 protein. (C) Apoptosis analysis (error bars are mean ±SD from 3 independent experiments). (D) Western blotting was performed with specific antibodies 72h after transfection with 5nM siC or siR2 in Tu212 and Bcl-2 overexpressing Tu212 (Tu212/Bcl-2) cell lines.
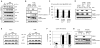
Apoptosis induction by knockdown of RRM2 is p53-, p73-, and Akt-independent. (A) Western blotting for p53 and p73 expression in Tu212 and A549 cells 72h after transfection with siC or siR2. (B) Western blotting for the indicated proteins in A549 and p53-knockdown A549 (A549/sh p53) cell lines after transfection with 5nM siC or siR2. A representative blot of three independent experiments is presented. (C) Apoptosis analysis (error bars are mean ±SD from 3 independent experiments). (D) Western blot analysis 72h after transfection with 5nM of siC or siR2 in H1299 and two clones of dominant negative p73-expressing H1299 (H1299/dN p73 Cl-7, Cl-10) cell lines. (E) Western blotting for p-Akt and Akt in Tu212 and A549 cells 72h after transfection with siC or different concentrations of siR2. (F) Apoptosis analysis. (G) Western blot of whole cell lysates from A549 and Akt-overexpressing A549 (A549/Akt) cell lines 72 h post-siRNA transfection.
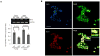
RRM2 regulates Bcl-2 through direct protein-protein interaction and partially influences Bcl-2 mRNA. (A) RT-PCR for Bcl-2 and GAPDH mRNA levels 72 h after transfection of Tu212 and A549 cells with 5nM siC or siR2. RRM2 was normalized to GAPDH levels within the same sample. (B) Tu212 cells were stained with anti-RRM2 (green), anti-Bcl-2 (red) and nuclei were counter stained with DAPI (blue). A z-stack of optical sections was created at 0.59 um intervals using a confocal microscope (LSM 510; Carl Zeiss MicroImaging, Inc.). Inset, upper panel shows magnification of white box in merged figure. Inset, lower panel shows z-axis reconstructions along the bars indicated in inset upper panel. Bar, 50μm. (C) Tu212 and (D) A549 cells were stained with anti-RRM2 (green), anti-Bcl-2 (red) and nuclei (blue) 48hr after transfection with 5 nM siC or siR2. Bar, 50μm. (E) Co-IP with anti-RRM2 (left panel) or anti-Bcl-2 (right panel) and immunoblotting with anti-Bcl-2 or anti-RRM2 in Tu212 and A549 cells.
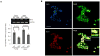
RRM2 regulates Bcl-2 through direct protein-protein interaction and partially influences Bcl-2 mRNA. (A) RT-PCR for Bcl-2 and GAPDH mRNA levels 72 h after transfection of Tu212 and A549 cells with 5nM siC or siR2. RRM2 was normalized to GAPDH levels within the same sample. (B) Tu212 cells were stained with anti-RRM2 (green), anti-Bcl-2 (red) and nuclei were counter stained with DAPI (blue). A z-stack of optical sections was created at 0.59 um intervals using a confocal microscope (LSM 510; Carl Zeiss MicroImaging, Inc.). Inset, upper panel shows magnification of white box in merged figure. Inset, lower panel shows z-axis reconstructions along the bars indicated in inset upper panel. Bar, 50μm. (C) Tu212 and (D) A549 cells were stained with anti-RRM2 (green), anti-Bcl-2 (red) and nuclei (blue) 48hr after transfection with 5 nM siC or siR2. Bar, 50μm. (E) Co-IP with anti-RRM2 (left panel) or anti-Bcl-2 (right panel) and immunoblotting with anti-Bcl-2 or anti-RRM2 in Tu212 and A549 cells.
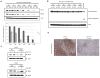
Depletion of RRM2 reduces Bcl-2 protein expression and regulates protein stability. (A, B) Tu212 cells were transfected with 5 nM siC or siR2, reseeded after 24h into 60-mm dishes and 24h later treated with cyclohexamide (100 μg/ml) for 0, 1, 3, 6, 9 and 12h. Cell lysates were collected at indicated time points and Western blotting performed with specific antibodies. (C) Tu212 and A549 cells were transfected with 5nM siC or siR2 and 24 hours later treated with 10 μmol/L MG132 for 2 hours before analyzing cell lysates by Western blotting. (D) Bcl-2 expression was detected in xenograft tumor tissue by IHC analysis. The animal study was conducted previously by treating with four doses of siCON1 (control nanoparticle) or CALAA-01 (RRM2 siRNA-nanoparticle). Representative images shown from siCON1 and CALAA-01 10 mg/kg groups (brown stain for Bcl-2 and nuclei were counter-stained by hematoxylin, blue; magnification X200).
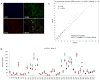
RRM2 and Bcl-2 proteins co-localize and their expression is positively correlated in tumor tissues from HNSCC and NSCLC patients. (A and D) Staining of RRM2 and Bcl-2 in paraffin-embedded formalin-fixed HNSCC (A) and NSCLC (D) tissue sections using primary antibodies with QD-secondary antibody conjugates. A representative QD-image is shown (400X magnification). (B and E) Quantification of QD signals. Average signals of RRM2 and Bcl-2 expression in each HNSCC (B) and NSCLC (E) patient sample were plotted. (C and F) Spearman’s correlation coefficient was estimated between Bcl-2 and RRM2 levels in HNSCC (C) and NSCLC (F) tumors. A linear regression was used to plot their relationship with 95% confidence interval bound.
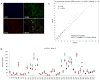
RRM2 and Bcl-2 proteins co-localize and their expression is positively correlated in tumor tissues from HNSCC and NSCLC patients. (A and D) Staining of RRM2 and Bcl-2 in paraffin-embedded formalin-fixed HNSCC (A) and NSCLC (D) tissue sections using primary antibodies with QD-secondary antibody conjugates. A representative QD-image is shown (400X magnification). (B and E) Quantification of QD signals. Average signals of RRM2 and Bcl-2 expression in each HNSCC (B) and NSCLC (E) patient sample were plotted. (C and F) Spearman’s correlation coefficient was estimated between Bcl-2 and RRM2 levels in HNSCC (C) and NSCLC (F) tumors. A linear regression was used to plot their relationship with 95% confidence interval bound.
Similar articles
-
Lu H, Yang X, Duggal P, Allen CT, Yan B, Cohen J, Nottingham L, Romano RA, Sinha S, King KE, Weinberg WC, Chen Z, Van Waes C. Lu H, et al. Cancer Res. 2011 Nov 1;71(21):6867-77. doi: 10.1158/0008-5472.CAN-11-2460. Epub 2011 Sep 20. Cancer Res. 2011. PMID: 21933882 Free PMC article.
-
Systemic delivery of siRNA nanoparticles targeting RRM2 suppresses head and neck tumor growth.
Rahman MA, Amin AR, Wang X, Zuckerman JE, Choi CH, Zhou B, Wang D, Nannapaneni S, Koenig L, Chen Z, Chen ZG, Yen Y, Davis ME, Shin DM. Rahman MA, et al. J Control Release. 2012 May 10;159(3):384-92. doi: 10.1016/j.jconrel.2012.01.045. Epub 2012 Feb 8. J Control Release. 2012. PMID: 22342644 Free PMC article.
-
Liu J, Uematsu H, Tsuchida N, Ikeda MA. Liu J, et al. Mol Cancer. 2011 Jul 31;10:95. doi: 10.1186/1476-4598-10-95. Mol Cancer. 2011. PMID: 21801448 Free PMC article.
-
Cancer Fighting SiRNA-RRM2 Loaded Nanorobots.
Sharma A, Kumar P, Ambasta RK. Sharma A, et al. Pharm Nanotechnol. 2020;8(2):79-90. doi: 10.2174/2211738508666200128120142. Pharm Nanotechnol. 2020. PMID: 32003677 Review.
-
Molecular genetics of head and neck cancer (Review).
Arora S, Aggarwal P, Pathak A, Bhandari R, Duffoo F, Gulati SC. Arora S, et al. Mol Med Rep. 2012 Jul;6(1):19-22. doi: 10.3892/mmr.2012.889. Epub 2012 Apr 24. Mol Med Rep. 2012. PMID: 22552728 Review.
Cited by
-
Interpreting pathways to discover cancer driver genes with Moonlight.
Colaprico A, Olsen C, Bailey MH, Odom GJ, Terkelsen T, Silva TC, Olsen AV, Cantini L, Zinovyev A, Barillot E, Noushmehr H, Bertoli G, Castiglioni I, Cava C, Bontempi G, Chen XS, Papaleo E. Colaprico A, et al. Nat Commun. 2020 Jan 3;11(1):69. doi: 10.1038/s41467-019-13803-0. Nat Commun. 2020. PMID: 31900418 Free PMC article.
-
Curcumin induces apoptosis of upper aerodigestive tract cancer cells by targeting multiple pathways.
Amin AR, Haque A, Rahman MA, Chen ZG, Khuri FR, Shin DM. Amin AR, et al. PLoS One. 2015 Apr 24;10(4):e0124218. doi: 10.1371/journal.pone.0124218. eCollection 2015. PLoS One. 2015. PMID: 25910231 Free PMC article.
-
Ciribilli Y, Singh P, Inga A, Borlak J. Ciribilli Y, et al. Oncotarget. 2016 Oct 4;7(40):65514-65539. doi: 10.18632/oncotarget.11804. Oncotarget. 2016. PMID: 27602772 Free PMC article.
-
Yin J, He X, Xia H, He L, Li D, Hu L, Zheng S, Huang Y, Li S, Hu W. Yin J, et al. Front Surg. 2022 Feb 3;9:821600. doi: 10.3389/fsurg.2022.821600. eCollection 2022. Front Surg. 2022. PMID: 35187059 Free PMC article.
References
-
- Engstrom Y, Eriksson S, Jildevik I, Skog S, Thelander L, Tribukait B. Cell cycle-dependent expression of mammalian ribonucleotide reductase. Differential regulation of the two subunits. J Biol Chem. 1985;260:9114–6. - PubMed
-
- Duxbury MS, Ito H, Zinner MJ, Ashley SW, Whang EE. RNA interference targeting the M2 subunit of ribonucleotide reductase enhances pancreatic adenocarcinoma chemosensitivity to gemcitabine. Oncogene. 2004;23:1539–48. - PubMed
Publication types
MeSH terms
Substances
LinkOut - more resources
Full Text Sources
Other Literature Sources
Medical
Miscellaneous