Relationship between size summation properties, contrast sensitivity and response latency in the dorsomedial and middle temporal areas of the primate extrastriate cortex - PubMed
- ️Tue Jan 01 2013
Relationship between size summation properties, contrast sensitivity and response latency in the dorsomedial and middle temporal areas of the primate extrastriate cortex
Leo L Lui et al. PLoS One. 2013.
Abstract
Analysis of the physiological properties of single neurons in visual cortex has demonstrated that both the extent of their receptive fields and the latency of their responses depend on stimulus contrast. Here, we explore the question of whether there are also systematic relationships between these response properties across different cells in a neuronal population. Single unit recordings were obtained from the middle temporal (MT) and dorsomedial (DM) extrastriate areas of anaesthetized marmoset monkeys. For each cell, spatial integration properties (length and width summation, as well as the presence of end- and side-inhibition within 15° of the receptive field centre) were determined using gratings of optimal direction of motion and spatial and temporal frequencies, at 60% contrast. Following this, contrast sensitivity was assessed using gratings of near-optimal length and width. In both areas, we found a relationship between spatial integration and contrast sensitivity properties: cells that summated over smaller areas of the visual field, and cells that displayed response inhibition at larger stimulus sizes, tended to show higher contrast sensitivity. In a sample of MT neurons, we found that cells showing longer latency responses also tended to summate over larger expanses of visual space in comparison with neurons that had shorter latencies. In addition, longer-latency neurons also tended to show less obvious surround inhibition. Interestingly, all of these effects were stronger and more consistent with respect to the selectivity for stimulus width and strength of side-inhibition than for length selectivity and end-inhibition. The results are partially consistent with a hierarchical model whereby more extensive receptive fields require convergence of information from larger pools of "feedforward" afferent neurons to reach near-optimal responses. They also suggest that a common gain normalization mechanism within MT and DM is involved, the spatial extent of which is more evident along the cell's preferred axis of motion.
Conflict of interest statement
Competing Interests: The authors have declared that no competing interests exist.
Figures
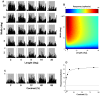
(A) Shows the matrix of PSTHs representing trials to all lengths and widths presented. The vertical scale, displaying response rate in spike/sec is located on the bottom left plot; the same scale applies to all histograms. (B) Shows the optimal fit of equation (1), which estimates response with respect to length and width. The arrows indicate estimates of optimal length and width, given by fitted parameters. This neuron is end-inhibited (EI) but shows no evidence of side inhibition when probed with stimuli covering up to 30° of visual angle (NSI15). (C) Displays peri-stimulus time histograms representing response over time to gratings of varying contrasts, shown with the same conversions as in (A). (D) Show the mean responses with respect to varying contrasts over the entire 2 sec presentation, fitted with equation (4). The arrow indicates C50. Thin grey line indicates mean spontaneous activity measured in the 500 ms before the onset of stimuli.
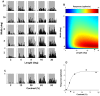
(A) Shows the matrix of PSTHs obtained by presentation of all lengths and widths tested, and (B) the shows the optimal fit of equation (1), with estimates of optimal length and width (arrows). This neuron is side-inhibited (SI) but shows no evidence of end inhibition when probed with stimuli covering up to 30° of visual angle (NEI15). (C) Displays PSTHs representing response over time to gratings of varying contrasts. (D) Show the mean responses with respect to varying contrasts, and an estimate of C50 (arrow).
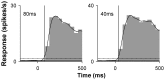
For each example, grey bars demonstrate the “grand PSTHs” (representing the neuron’s response to gratings of optimal orientation, spatial and temporal frequency, and various sizes), with respect to stimulus onset (0 ms). The curved line illustrates the spike density function for which the latency was calculated. The solid horizontal line denotes the average spontaneous activity while the dotted line is one standard deviation above the spontaneous activity. The time at which these spike density function crosses this threshold represents an estimate of the latency of a particular cell (indicated by the vertical line and number). Both examples were presented via the “flashed” method.
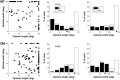
Left column: the preferred length and width for each cell. Comparison of the distribution of optimal lengths (middle column) and optimal widths (right column) are also shown. For all histogram arrows denote the median of each distribution. DM cells prefer significantly longer gratings while MT cells prefer wider gratings (p<0.05).
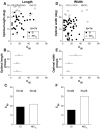
Separate analyses are presented for the length (left column) and width (right column) dimensions of the receptive field. Top row shows the relationship between the optimal length (A) and width (D) of the stimulus, and contrast sensitivity (C50). Data from cells that showed significant spatial inhibition upon presentation of stimuli up to 30° in length or width are indicated by filled circles (end inhibition in panel A, side inhibition in panel D). The middle row summarizes the data shown in the top row, by grouping neurons according to the preferred length (B) and width (E) in three groups, according to optimal size (<10°, 10–20° and >20°). The data points are medians for these groups, and error bars represent the inter quartile ranges. Bottom row illustrates the mean C50 for DM cells. Black bars represent means for EI and SI cells (in C and F, respectively), and white bars represent the means for NEI15 and NSI15 cells.
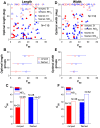
Top row shows the relationship between optimal length (A), width (D) and C50, with filled symbols indicating cells that displayed significant end and side-inhibition (in A and D, respectively) upon presentation of stimuli up to 30° in size. Results from cells tested with “flashed” gratings are indicated by blue triangles, and those from cells tested with “ramped” gratings by red circles. The middle row summarizes the data shown in the top row, by grouping neurons according to the preferred length (B) and width (E), in three groups according to optimal size (<10°, 10–20° and >20°). The data points are medians for these groups, and error bars represent inter quartile ranges. Bottom row illustrates the mean C50 for cells in MT, grouped according to their method of presentation and spatial inhibition properties along the length (C) and width (F) dimensions of the receptive field.
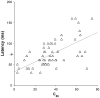
Only data using the “flashed” method of presentation is included here. Arrow indicates mean latency and line indicates the best linear fit.
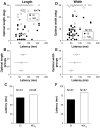
Top row shows the relationship between optimal length (A), width (D) and latency, with filled symbols indicating cells that displayed significant end and side-inhibition (in A and D, respectively) upon presentation of stimuli up to 30° in size. The middle row summarizes the data shown in the top row, by grouping neurons according to the preferred length (B) and width (E), in three groups according to optimal size (<10°, 10–20° and >20°). The data points are medians for these groups, and error bars represent inter quartile ranges. Bottom row illustrates the mean latency for cells in MT, grouped according to spatial inhibition properties along the length (C) and width (F) dimensions of the receptive field. Only data using the “flashed” method of presentation is included here.
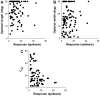
Top row illustrates the relationship between response and (A) optimal length and (B) optimal width. (C) Illustrates the relationship between response and C50 for DM.

Top row illustrates the relationship between response and (A) optimal length and (B) optimal width. The method of presentation is identified, see legend in (A). (C) Shows the relationship between response and C50while (D) illustrates the relationship between response and latency for MT.
Similar articles
-
Receptive-field properties of neurons in middle temporal visual area (MT) of owl monkeys.
Felleman DJ, Kaas JH. Felleman DJ, et al. J Neurophysiol. 1984 Sep;52(3):488-513. doi: 10.1152/jn.1984.52.3.488. J Neurophysiol. 1984. PMID: 6481441
-
Receptive fields and functional architecture of macaque V2.
Levitt JB, Kiper DC, Movshon JA. Levitt JB, et al. J Neurophysiol. 1994 Jun;71(6):2517-42. doi: 10.1152/jn.1994.71.6.2517. J Neurophysiol. 1994. PMID: 7931532
-
Response latencies of neurons in visual areas MT and MST of monkeys with striate cortex lesions.
Azzopardi P, Fallah M, Gross CG, Rodman HR. Azzopardi P, et al. Neuropsychologia. 2003;41(13):1738-56. doi: 10.1016/s0028-3932(03)00176-3. Neuropsychologia. 2003. PMID: 14527538
-
Contextual modulation and stimulus selectivity in extrastriate cortex.
Krause MR, Pack CC. Krause MR, et al. Vision Res. 2014 Nov;104:36-46. doi: 10.1016/j.visres.2014.10.006. Epub 2014 Nov 3. Vision Res. 2014. PMID: 25449337 Review.
Cited by
-
A simpler primate brain: the visual system of the marmoset monkey.
Solomon SG, Rosa MG. Solomon SG, et al. Front Neural Circuits. 2014 Aug 8;8:96. doi: 10.3389/fncir.2014.00096. eCollection 2014. Front Neural Circuits. 2014. PMID: 25152716 Free PMC article. Review.
-
Interocular contrast difference drives illusory 3D percept.
Reynaud A, Hess RF. Reynaud A, et al. Sci Rep. 2017 Jul 17;7(1):5587. doi: 10.1038/s41598-017-06151-w. Sci Rep. 2017. PMID: 28717190 Free PMC article.
-
Khazaei S, Faghih RT. Khazaei S, et al. PNAS Nexus. 2024 Aug 28;3(9):pgae370. doi: 10.1093/pnasnexus/pgae370. eCollection 2024 Sep. PNAS Nexus. 2024. PMID: 39282005 Free PMC article.
-
An Unexpected Spontaneous Motion-In-Depth Pulfrich Phenomenon in Amblyopia.
Reynaud A, Hess RF. Reynaud A, et al. Vision (Basel). 2019 Oct 22;3(4):54. doi: 10.3390/vision3040054. Vision (Basel). 2019. PMID: 31735855 Free PMC article.
-
Functional MRI of visual responses in the awake, behaving marmoset.
Hung CC, Yen CC, Ciuchta JL, Papoti D, Bock NA, Leopold DA, Silva AC. Hung CC, et al. Neuroimage. 2015 Oct 15;120:1-11. doi: 10.1016/j.neuroimage.2015.06.090. Epub 2015 Jul 3. Neuroimage. 2015. PMID: 26149609 Free PMC article.
References
-
- Levitt JB, Lund JS (1997) Contrast dependence of contextual effects in primate visual cortex. Nature 387: 73–76. - PubMed
-
- Sceniak MP, Ringach DL, Hawken MJ, Shapley R (1999) Contrast’s effect on spatial summation by macaque V1 neurons. Nat Neurosci 2: 733–739. - PubMed
-
- Cavanaugh JR, Bair W, Movshon JA (2002) Selectivity and spatial distribution of signals from the receptive field surround in macaque V1 neurons. J Neurophysiol 88: 2547–2556. - PubMed
-
- Pack CC, Hunter JN, Born RT (2005) Contrast dependence of suppressive influences in cortical area MT of alert macaque. J Neurophysiol 93: 1809–1815. - PubMed
Publication types
MeSH terms
Grants and funding
This work was funded by the Australian Research Council, www.arc.gov.au, (Grant DP0878965); Bionic Vision Sciences and Technology Initiative (SR100006); and by the National Health and Medical Research Council, www.nhmrc.gov.au, (Grant 491022). LL was funded by CJ Martin Biomedical Fellowship (490908) awarded by the National Health and Medical Council of Australia. The funders had no role in study design, data collection and analysis, decision to publish, or preparation of the manuscript.
LinkOut - more resources
Full Text Sources
Other Literature Sources