Cohesin-mediated interactions organize chromosomal domain architecture - PubMed
- ️Tue Jan 01 2013
Cohesin-mediated interactions organize chromosomal domain architecture
Sevil Sofueva et al. EMBO J. 2013.
Abstract
To ensure proper gene regulation within constrained nuclear space, chromosomes facilitate access to transcribed regions, while compactly packaging all other information. Recent studies revealed that chromosomes are organized into megabase-scale domains that demarcate active and inactive genetic elements, suggesting that compartmentalization is important for genome function. Here, we show that very specific long-range interactions are anchored by cohesin/CTCF sites, but not cohesin-only or CTCF-only sites, to form a hierarchy of chromosomal loops. These loops demarcate topological domains and form intricate internal structures within them. Post-mitotic nuclei deficient for functional cohesin exhibit global architectural changes associated with loss of cohesin/CTCF contacts and relaxation of topological domains. Transcriptional analysis shows that this cohesin-dependent perturbation of domain organization leads to widespread gene deregulation of both cohesin-bound and non-bound genes. Our data thereby support a role for cohesin in the global organization of domain structure and suggest that domains function to stabilize the transcriptional programmes within them.
Figures
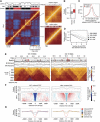
Cohesin/CTCF sites anchor chromatin loops of multiple sizes. (A) Hi-C contact map of a 70-Mb region on chromosome 1 in NSCs, coloured according to technically corrected contact enrichment (Yaffe and Tanay, 2011). Active chromatin (red cluster) is enriched for transcription start sites (TSSs) and depleted for lamina interactions, whereas passive chromatin (black cluster) exhibits the opposite characteristics (see also Supplementary Figure S7). Insets: zoomed-in active and passive regions. (B) Genome-wide distributions of contact enrichment within active and passive domains, for genomic distances between 60 and 180 Kb (log2 scale). (C) Distribution of the distance between adjacent cohesin binding sites within active and passive domains (Kolmogorov–Smirnov D=0.1924, P<<10−10). (D) Pairs of elements were grouped according to the number of binding sites separating them (x axis) and their genomic distance. The average contact enrichment is shown, relative to the group genomic average (y axis, log2 scale). (E) Hi-C contact maps of a 3-Mb region on chromosomes 18 and 10 in NSCs (the matrix has been rotated by 45 degrees). Rad21 binding sites and TSSs are shown. Average contact enrichments represent the intensity of interactions crossing each genomic locus, while controlling for genomic distance (tracks for the 80 or 640 Kb bands are shown). (F) Multi-scale colour-coded heatmaps of the average contact intensity around cohesin/CTCF binding sites, using a series of high-resolution bands ranging from 10 to 80 Kb in both AST and NSCs. (G) Left panel, comparison of the average contact intensity for Rad21/CTCF sites (red) with that for active genes (blue) and silent genes (black) at the 80-Kb band and right panel, with that for CTCF-only (yellow) or Rad21-only (green) sites.
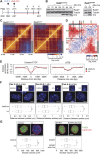
Loss of a functional cohesin complex perturbs nuclear structure. (A) Differentiation scheme of Rad21Lox/Lox NSCs to Rad21Δ/Δ ASTs. NSCs were grown in the presence of EGF/FGF (dotted line) followed by replacement with BMP4 (blue arrow). Twenty-four hours later, ASTs were infected with Adv-Cre (red arrow). Grey arrowheads represent sample collection time points. (B) Immunoblot analysis of changes in Rad21 protein levels following Adv-Cre addition to Rad21Lox/Lox and Rad21WT/WT AST cultures. Eighty-nine percent of the Rad21 protein is lost 96 h after infection specifically in Rad21Lox/Lox cells (quantified with ImageJ). (C) Hi-C contact maps of a 6-Mb region on chr 1 from Rad21Lox/Lox and Rad21Δ/Δ AST cells. (D) A delta contact map colour-coded according to the difference in normalized contact intensity between the two conditions in (C). Indicated are the locations of 3D DNA FISH probes (A–E) using colour bars, probes have been paired on the matrix using straight lines. (E) Average contact intensities (80 Kb band) across cohesin/CTCF binding sites and aTSSs, comparing Rad21Lox/Lox ASTs (black) to Rad21Δ/Δ ASTs (red). (F) Representative confocal images of nuclei and quantification of three-dimensional inter-probe distances for the indicated probe pairs in Rad21Lox/Lox and Rad21Δ/Δ ASTs (Volocity software). DNA has been counterstained with DAPI (blue) and probes are labelled with DIG (green) or biotin (red). Genomic distances between probes are indicated. White boxes show the regions that have been zoomed in. Whiskers and boxes indicate all and 50% of values, respectively. Central bold bars represent the median. The asterisk indicates a statistically significant difference as assessed using a Wilcoxon Rank-Sum and a Signed-Rank Test (medians: pair C–D 0.30/0.34 microns, P=0.01; pair B–D 0.49/0.55 microns, P=0.04; pair A–E 0.87/0.75 microns, P=0.009). (G) Maximum projections from confocal z-stacks of Rad21Lox/Lox and Rad21WT/WT AST nuclei treated with or without Adv-Cre and stained for Rad21 (green) and Lamin A/C (red). DNA has been counterstained with DAPI (blue). Quantifications and statistical analysis were done as above. Median nuclear volumes −334 (Lox/Lox) versus 422 (Δ/Δ), P=1.3 × 10−10 and 412 (WT/WT) versus 445 (WT/WT+Adv-Cre), P=0.04. Each experiment was repeated a minimum of two times (n>170/condition). Scale bar=5 μm. *P>0.01, **P<0.01.
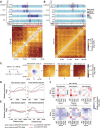
Cohesins engage in preferential long-range interactions. (A) Results from 4C-seq experiments for two viewpoints (black dots) located at cohesin/CTCF binding sites proximal to a border between two active domains, and (B) two viewpoints located at a cohesin/CTCF site (right bait) and a cohesin site within a large passive domain or proximal to its border. Each 4C-seq experiment is represented by the median normalized 4C-seq coverage in a sliding window of 5 Kb (top) and a multi-scale domainogram indicating normalized mean coverage in windows ranging between 2 and 50 Kb. Rad21, CTCF binding profiles and the corresponding Hi-C submatrices are also shown (bottom). (C) We pooled together Hi-C submatrices, aligned over an interaction between two cohesin binding sites (centre point) and computed the average contact enrichment in high-resolution bins of 2 Kb. Shown are matrices representing interactions between 6058 pairs of cohesin sites that are located in the same domain (intra-domain) and 2771 pairs of cohesin sites located in distinct domains (inter-domain), in both control and Rad21-deficient cells, in all cases controlling for genomic distance (100–200 Kb). Note the specific colour-coding scheme used, designed to highlight the Hi-C dynamic range at these genomic distances. (D) Relative intra-domain contact enrichment as a function of distance, when the two sites are <5 Kb away from a cohesin/CTCF binding site (black) or where only one site is <5 Kb away from a cohesin/CTCF site (dashed black). Data for Rad21-deficient cells (red and dashed red) are also shown. (E) Same as in (D) but showing inter-domain contacts. (F) Same as the analysis in (D, E) but now testing preferential contacts between different classes of genomic loci; cohesin/CTCF sites (COH), cohesin-free CTCF sites (CTCF), active and silent TSSs (aTSS and sTSS, respectively) and putative enhancers based on p300 binding (ENH). Shown are enrichment values computed for intra-domain contacts at genomic distances of 100–160 Kb (leftmost panels), and for inter-domain contacts at genomic distances of 1–1.6 Mb, further classified to interactions between elements within active or passive domains (right).
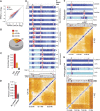
Large-scale transcriptional deregulation in cohesin-deficient cells. (A) Scatter plot comparison between the transcription level of genes in control cells (x axis) and cohesin-deficient cells (y axis). Genes were classified into 770 upregulated genes (z-score>2, red), 992 downregulated genes (z-score<2, blue) and minimal-change genes (grey). (B) Distribution of 1762 deregulated genes according to cohesin/CTCF occupancy at the TSS (<1 Kb), near the TSS (<10 Kb) and away from TSS (>10 Kb). (C) Enrichment of the number of deregulated genes in the groups defined in (B), over a background composed of all genes. Deregulated genes with cohesin/CTCF at the TSS are enriched by 44%. (D) Pearson correlation of the transcriptional response to cohesin knockout of gene pairs, which are 100–200 Kb apart and have no separating cohesin/CTCF site (red). The correlation for pairs of genes which are separated by at least one site is shown as a control (grey). (E) 4C-Seq viewpoints positioned (from left to right) at a cohesin/CTCF site 15 Kb upstream of the Deptor TSS, 830 bp from the Deptor TSS or 1.4 Kb upstream of the Col14a1 TSS. Shown are the 4C-Seq profiles during a time course of Rad21 deletion for each viewpoint, which reveal a progressive loss of cohesin–cohesin contacts with decreasing cohesin protein levels. Shown is the % drop in Rad21 protein levels at each time point based on a quantitative western blot analysis. (F) 4C-Seq viewpoints positioned 580 bp from the TSS of the downregulated Olfml3 gene (right bait) as well as at a Rad21/CTCF binding site 300 Kb away. These sites interact according to the Hi-C data and this interaction is specifically lost in Rad21-deficient cells. (G) 4C-Seq viewpoints positioned 3.1 Kb from the TSS of the upregulated Igfbp5 gene (right bait) and a cohesin/CTCF site 10 Kb away from the TSS. The latter preferentially interacts with the cohesin/CTCF site at the other edge of this large domain. This interaction is lost in the mutant. The ChIP-Seq tracks for Rad21, CTCF and TSS locations and change in expression are also shown.
Comment in
-
Cohesin's role as an active chromatin domain anchorage revealed.
Feig C, Odom DT. Feig C, et al. EMBO J. 2013 Dec 11;32(24):3114-5. doi: 10.1038/emboj.2013.248. Epub 2013 Nov 22. EMBO J. 2013. PMID: 24270571 Free PMC article.
Similar articles
-
Cohesin and CTCF differentially affect chromatin architecture and gene expression in human cells.
Zuin J, Dixon JR, van der Reijden MI, Ye Z, Kolovos P, Brouwer RW, van de Corput MP, van de Werken HJ, Knoch TA, van IJcken WF, Grosveld FG, Ren B, Wendt KS. Zuin J, et al. Proc Natl Acad Sci U S A. 2014 Jan 21;111(3):996-1001. doi: 10.1073/pnas.1317788111. Epub 2013 Dec 13. Proc Natl Acad Sci U S A. 2014. PMID: 24335803 Free PMC article.
-
Degner SC, Verma-Gaur J, Wong TP, Bossen C, Iverson GM, Torkamani A, Vettermann C, Lin YC, Ju Z, Schulz D, Murre CS, Birshtein BK, Schork NJ, Schlissel MS, Riblet R, Murre C, Feeney AJ. Degner SC, et al. Proc Natl Acad Sci U S A. 2011 Jun 7;108(23):9566-71. doi: 10.1073/pnas.1019391108. Epub 2011 May 23. Proc Natl Acad Sci U S A. 2011. PMID: 21606361 Free PMC article.
-
Cohesin is positioned in mammalian genomes by transcription, CTCF and Wapl.
Busslinger GA, Stocsits RR, van der Lelij P, Axelsson E, Tedeschi A, Galjart N, Peters JM. Busslinger GA, et al. Nature. 2017 Apr 27;544(7651):503-507. doi: 10.1038/nature22063. Epub 2017 Apr 19. Nature. 2017. PMID: 28424523 Free PMC article.
-
Genetic Tailors: CTCF and Cohesin Shape the Genome During Evolution.
Vietri Rudan M, Hadjur S. Vietri Rudan M, et al. Trends Genet. 2015 Nov;31(11):651-660. doi: 10.1016/j.tig.2015.09.004. Epub 2015 Oct 1. Trends Genet. 2015. PMID: 26439501 Review.
-
Lee BK, Iyer VR. Lee BK, et al. J Biol Chem. 2012 Sep 7;287(37):30906-13. doi: 10.1074/jbc.R111.324962. Epub 2012 Sep 5. J Biol Chem. 2012. PMID: 22952237 Free PMC article. Review.
Cited by
-
Chromosomes at Work: Organization of Chromosome Territories in the Interphase Nucleus.
Fritz AJ, Barutcu AR, Martin-Buley L, van Wijnen AJ, Zaidi SK, Imbalzano AN, Lian JB, Stein JL, Stein GS. Fritz AJ, et al. J Cell Biochem. 2016 Jan;117(1):9-19. doi: 10.1002/jcb.25280. J Cell Biochem. 2016. PMID: 26192137 Free PMC article.
-
Li R, Liu Y, Li T, Li C. Li R, et al. Sci Rep. 2016 Oct 13;6:34651. doi: 10.1038/srep34651. Sci Rep. 2016. PMID: 27734896 Free PMC article.
-
Groves IJ, O'Connor CM. Groves IJ, et al. J Virol. 2024 Oct 22;98(10):e0114824. doi: 10.1128/jvi.01148-24. Epub 2024 Aug 30. J Virol. 2024. PMID: 39212383 Review.
-
Gelot C, Guirouilh-Barbat J, Lopez BS. Gelot C, et al. Nucleus. 2016 Jul 3;7(4):339-45. doi: 10.1080/19491034.2016.1194159. Epub 2016 Jun 21. Nucleus. 2016. PMID: 27326661 Free PMC article. Review.
-
Pezic D, Weeks S, Varsally W, Dewari PS, Pollard S, Branco MR, Hadjur S. Pezic D, et al. Stem Cell Reports. 2023 Nov 14;18(11):2154-2173. doi: 10.1016/j.stemcr.2023.09.004. Epub 2023 Oct 5. Stem Cell Reports. 2023. PMID: 37802073 Free PMC article.
References
-
- Andrey G, Montavon T, Mascrez B, Gonzalez F, Noordermeer D, Leleu M, Trono D, Spitz F, Duboule D. A switch between topological domains underlies HoxD genes collinearity in mouse limbs. Science. 2013;340:1234167–1234167. - PubMed
-
- Ciosk R, Shirayama M, Shevchenko A, Tanaka T, Toth A, Shevchenko A, Nasmyth K. Cohesin's binding to chromosomes depends on a separate complex consisting of Scc2 and Scc4 proteins. Mol Cell. 2000;5:243–254. - PubMed
-
- Degner SC, Verma-Gaur J, Wong TP, Bossen C, Iverson GM, Torkamani A, Vettermann C, Lin YC, Ju Z, Schulz D, Murre CS, Birshtein BK, Schork NJ, Schlissel MS, Riblet R, Murre C, Feeney AJ. CCCTC-binding factor (CTCF) and cohesin influence the genomic architecture of the Igh locus and antisense transcription in pro-B cells. Proc Natl Acad Sci USA. 2011;108:9566–9571. - PMC - PubMed
Publication types
MeSH terms
Substances
LinkOut - more resources
Full Text Sources
Other Literature Sources
Molecular Biology Databases