Microbiome of prebiotic-treated mice reveals novel targets involved in host response during obesity - PubMed
Microbiome of prebiotic-treated mice reveals novel targets involved in host response during obesity
Amandine Everard et al. ISME J. 2014 Oct.
Abstract
The gut microbiota is involved in metabolic and immune disorders associated with obesity and type 2 diabetes. We previously demonstrated that prebiotic treatment may significantly improve host health by modulating bacterial species related to the improvement of gut endocrine, barrier and immune functions. An analysis of the gut metagenome is needed to determine which bacterial functions and taxa are responsible for beneficial microbiota-host interactions upon nutritional intervention. We subjected mice to prebiotic (Pre) treatment under physiological (control diet: CT) and pathological conditions (high-fat diet: HFD) for 8 weeks and investigated the production of intestinal antimicrobial peptides and the gut microbiome. HFD feeding significantly decreased the expression of regenerating islet-derived 3-gamma (Reg3g) and phospholipase A2 group-II (PLA2g2) in the jejunum. Prebiotic treatment increased Reg3g expression (by ∼50-fold) and improved intestinal homeostasis as suggested by the increase in the expression of intectin, a key protein involved in intestinal epithelial cell turnover. Deep metagenomic sequencing analysis revealed that HFD and prebiotic treatment significantly affected the gut microbiome at different taxonomic levels. Functional analyses based on the occurrence of clusters of orthologous groups (COGs) of proteins also revealed distinct profiles for the HFD, Pre, HFD-Pre and CT groups. Finally, the gut microbiota modulations induced by prebiotics counteracted HFD-induced inflammation and related metabolic disorders. Thus, we identified novel putative taxa and metabolic functions that may contribute to the development of or protection against the metabolic alterations observed during HFD feeding and HFD-Pre feeding.
Figures
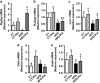
HFD feeding and prebiotic treatment affect antimicrobial peptides in the intestine. Antibacterial peptide mRNA expression: (a) Regenerating islet-derived 3-gamma (RegIIIγ, encoded by Reg3g); (b) Phospholipase A2 group II (encoded by Pla2g2); (c) Lysozyme C (encoded by Lyz1); (d) α-defensins (encoded by Defa); and (e) Angiogenin 4 (encoded by Ang4) measured in the jejunum of control diet-fed mice (CT) (n=9), CT diet-fed mice treated with prebiotics (CT-Pre) (n=10), HFD-fed mice (HFD) (n=10) and HFD-fed mice treated with prebiotics (HFD-Pre) (n=10). Data are means±s.e.m. Data with different superscript letters are significantly different (P<0.05) according to a post hoc ANOVA one-way statistical analysis.
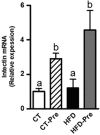
Prebiotic treatment increases colon intectin expression, a protein involved in the turnover of intestinal mucosa. Intectin mRNA expression measured in the colon of control diet-fed mice (CT) (n=9), CT diet-fed mice treated with prebiotics (CT-Pre) (n=10), HFD-fed mice (HFD) (n=10) and HFD-fed mice treated with prebiotics (HFD-Pre) (n=10). Data are means±s.e.m. Data with different superscript letters are significantly different (P<0.05) according to a post hoc ANOVA one-way statistical analysis.
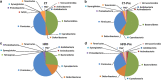
HFD feeding and prebiotic treatment affect the proportions of different phyla. The composition of abundant bacterial phyla identified in the gut microbiota of (a) control diet-fed mice (CT) (n=9), (b) CT diet-fed mice treated with prebiotics (CT-Pre) (n=9), (c) HFD-fed mice (HFD) (n=7) and (d) HFD-fed mice treated with prebiotics (HFD-Pre) (n=10). Undetected phyla are not represented on the pie chart. The significant changes in specific phyla are shown in Supplementary Table S1.
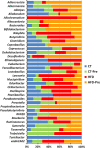
HFD feeding and prebiotic treatment affect the proportions of different genera. The composition of the bacterial genera significantly affected by the treatment and identified in the gut microbiota of control diet-fed mice (CT) (n=9), CT diet-fed mice treated with prebiotics (CT-Pre) (n=9), HFD-fed mice (HFD) (n=7) and HFD-fed mice treated with prebiotics (HFD-Pre) (n=10). Each column is set at 100% to illustrate the proportion of each genus among the different groups; the absence of any colour indicates that the genus was not detected in this group of mice. The statistically significant changes observed between different groups are shown in Supplementary Table S2.
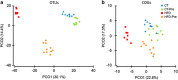
PCoA of the gut bacterial communities and COG abundance. The analysis was based on the Bray–Curtis similarity matrix constructed using the square-root-transformed OTU (a) or COG abundances (b). The per cent of variation explained is given in brackets. OTU, operational taxonomic unit.
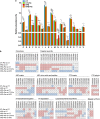
Orthologous groups of proteins (COGs) affected by the dietary treatments. (a) Occurrence of identified orthologous groups of proteins (COGs) according to COG functional categories: (A) RNA processing and modification are not shown on the figure since the abundance (%) was under 0.0025% in all four diet groups; (C) Energy production and conversion; (D) Cell-cycle control, cell division and chromosome partitioning; (E) Amino acid transport and metabolism; (F) Nucleotide transport and metabolism; (G) Carbohydrate transport and metabolism; (H) Coenzyme transport and metabolism; (I) Lipid transport and metabolism; (J) Translation, ribosomal structure and biogenesis; (K) Transcription; (L) Replication, recombination and repair; (M) Cell wall/membrane/envelope biogenesis; (N) Cell motility; (O) Posttranslational modification, protein turnover, chaperones; (P) Inorganic ion transport and metabolism; (Q) Secondary metabolites biosynthesis, transport and catabolism; (R) General function prediction only; (S) function unknown; (T), Signal transduction mechanisms; (U) Intracellular trafficking, secretion and vesicular transport; (V), defence mechanisms. Data are means±s.e.m. *P<0.05, **P<0.01, ***P<0.001 vs CT. (b) Changes in the occurrence of COGs according to the metabolic pathways affected following the different treatments. COGs with a median of ⩾4 (∼0.005% of the total number of COGs in normalised data sets) in at least one of the four diet groups were compared. Red and blue fields correspond to statistically significant increases and decreases in the COG relative abundances, respectively. The details of each COG number are shown in Supplementary Table S3.

Prebiotic treatment increases caecal short chain fatty acids content. (a) Actetate, (b) Propionate, (c) Butyrate, (d) Lactate and (e) Succinate concentrations (μmol g−1 of dry caecal content) measured in the caecal content of control diet-fed mice (CT) (n=9), CT diet-fed mice treated with prebiotics (CT-Pre) (n=10), HFD-fed mice (HFD) (n=10) and HFD-fed mice treated with prebiotics (HFD-Pre) (n=10). Data are means±s.e.m. Data with different superscript letters are significantly different (P<0.05) according to a post hoc ANOVA one-way statistical analysis.
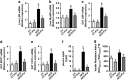
Prebiotic treatment decreased inflammation, fat mass development and insulin resistance associated with HFD-induced obesity. Inflammatory markers mRNA expression: (a) LPS binding protein (encoded by LBP) and (b) Myeloid differentiation primary response gene (88) (encoded by Myd88) in the liver; (c) LPS binding protein (encoded by LBP); (d) Monocyte chemoattractant protein 1 (encoded by MCP1) and (e) Cluster of differentiation 11c (encoded by CD11c) in the epididymal adipose tissue (EAT); (f) Plasma leptin (ng μl−1) in cava vein and (g) Insulin resistance index determined by multiplying the area under the curve (from 0 to 15 min) of blood glucose and plasma insulin following an oral glucose load (2 g glucose per kg of body weight) measured in control diet-fed mice (CT) (n=9), CT diet-fed mice treated with prebiotics (CT-Pre) (n=10), HFD-fed mice (HFD) (n=10) and HFD-fed mice treated with prebiotics (HFD-Pre) (n=10). Data are means±s.e.m. Data with different superscript letters are significantly different (P<0.05) according to a post hoc ANOVA one-way statistical analysis.
Similar articles
-
Cani PD, Possemiers S, Van de Wiele T, Guiot Y, Everard A, Rottier O, Geurts L, Naslain D, Neyrinck A, Lambert DM, Muccioli GG, Delzenne NM. Cani PD, et al. Gut. 2009 Aug;58(8):1091-103. doi: 10.1136/gut.2008.165886. Epub 2009 Feb 24. Gut. 2009. PMID: 19240062 Free PMC article.
-
Jiao X, Wang Y, Lin Y, Lang Y, Li E, Zhang X, Zhang Q, Feng Y, Meng X, Li B. Jiao X, et al. J Nutr Biochem. 2019 Feb;64:88-100. doi: 10.1016/j.jnutbio.2018.07.008. Epub 2018 Aug 11. J Nutr Biochem. 2019. PMID: 30471564
-
Everard A, Lazarevic V, Derrien M, Girard M, Muccioli GG, Neyrinck AM, Possemiers S, Van Holle A, François P, de Vos WM, Delzenne NM, Schrenzel J, Cani PD. Everard A, et al. Diabetes. 2011 Nov;60(11):2775-86. doi: 10.2337/db11-0227. Epub 2011 Sep 20. Diabetes. 2011. PMID: 21933985 Free PMC article.
-
Prebiotic effects: metabolic and health benefits.
Roberfroid M, Gibson GR, Hoyles L, McCartney AL, Rastall R, Rowland I, Wolvers D, Watzl B, Szajewska H, Stahl B, Guarner F, Respondek F, Whelan K, Coxam V, Davicco MJ, Léotoing L, Wittrant Y, Delzenne NM, Cani PD, Neyrinck AM, Meheust A. Roberfroid M, et al. Br J Nutr. 2010 Aug;104 Suppl 2:S1-63. doi: 10.1017/S0007114510003363. Br J Nutr. 2010. PMID: 20920376 Review.
-
Interplay between obesity and associated metabolic disorders: new insights into the gut microbiota.
Cani PD, Delzenne NM. Cani PD, et al. Curr Opin Pharmacol. 2009 Dec;9(6):737-43. doi: 10.1016/j.coph.2009.06.016. Epub 2009 Jul 21. Curr Opin Pharmacol. 2009. PMID: 19628432 Review.
Cited by
-
Puértolas-Balint F, Schroeder BO. Puértolas-Balint F, et al. Front Immunol. 2020 Jun 9;11:1164. doi: 10.3389/fimmu.2020.01164. eCollection 2020. Front Immunol. 2020. PMID: 32655555 Free PMC article. Review.
-
Cultivating healthy growth and nutrition through the gut microbiota.
Subramanian S, Blanton LV, Frese SA, Charbonneau M, Mills DA, Gordon JI. Subramanian S, et al. Cell. 2015 Mar 26;161(1):36-48. doi: 10.1016/j.cell.2015.03.013. Cell. 2015. PMID: 25815983 Free PMC article.
-
Omega-3-Rich Fish-Oil-Influenced Mouse Gut Microbiome Shaped by Intermittent Consumption of Beef.
Park J, Xuan B, Jeong Y, Han G, Kim EB. Park J, et al. Curr Microbiol. 2023 Mar 1;80(4):119. doi: 10.1007/s00284-023-03223-1. Curr Microbiol. 2023. PMID: 36855004
-
Natural diets promote retention of the native gut microbiota in captive rodents.
Martínez-Mota R, Kohl KD, Orr TJ, Denise Dearing M. Martínez-Mota R, et al. ISME J. 2020 Jan;14(1):67-78. doi: 10.1038/s41396-019-0497-6. Epub 2019 Sep 9. ISME J. 2020. PMID: 31495829 Free PMC article.
-
Gu C, Zhou Z, Yu Z, He M, He L, Luo Z, Xiao W, Yang Q, Zhao F, Li W, Shen L, Han J, Cao S, Zuo Z, Deng J, Yan Q, Ren Z, Zhao M, Yu S. Gu C, et al. Front Cell Infect Microbiol. 2022 May 27;12:870785. doi: 10.3389/fcimb.2022.870785. eCollection 2022. Front Cell Infect Microbiol. 2022. PMID: 35694542 Free PMC article.
References
-
- Altschul SF, Gish W, Miller W, Myers EW, Lipman DJ. Basic local alignment search tool. J Mol Biol. 1990;215:403–410. - PubMed
-
- Bevins CL, Salzman NH. Paneth cells, antimicrobial peptides and maintenance of intestinal homeostasis. Nat Rev Microbiol. 2011;9:356–368. - PubMed
-
- Cani PD, Amar J, Iglesias MA, Poggi M, Knauf C, Bastelica D, et al. Metabolic endotoxemia initiates obesity and insulin resistance. Diabetes. 2007;56:1761–1772. - PubMed
-
- Cani PD, Neyrinck AM, Fava F, Knauf C, Burcelin RG, Tuohy KM, et al. Selective increases of bifidobacteria in gut microflora improve high-fat-diet-induced diabetes in mice through a mechanism associated with endotoxaemia. Diabetologia. 2007;50:2374–2383. - PubMed
Publication types
MeSH terms
Substances
LinkOut - more resources
Full Text Sources
Other Literature Sources
Medical