Deciphering gamma-decalactone biosynthesis in strawberry fruit using a combination of genetic mapping, RNA-Seq and eQTL analyses - PubMed
- ️Wed Jan 01 2014
Deciphering gamma-decalactone biosynthesis in strawberry fruit using a combination of genetic mapping, RNA-Seq and eQTL analyses
José F Sánchez-Sevilla et al. BMC Genomics. 2014.
Abstract
Background: Understanding the basis for volatile organic compound (VOC) biosynthesis and regulation is of great importance for the genetic improvement of fruit flavor. Lactones constitute an essential group of fatty acid-derived VOCs conferring peach-like aroma to a number of fruits including peach, plum, pineapple and strawberry. Early studies on lactone biosynthesis suggest that several enzymatic pathways could be responsible for the diversity of lactones, but detailed information on them remained elusive. In this study, we have integrated genetic mapping and genome-wide transcriptome analysis to investigate the molecular basis of natural variation in γ-decalactone content in strawberry fruit.
Results: As a result, the fatty acid desaturase FaFAD1 was identified as the gene underlying the locus at LGIII-2 that controls γ-decalactone production in ripening fruit. The FaFAD1 gene is specifically expressed in ripe fruits and its expression fully correlates with the presence of γ-decalactone in all 95 individuals of the mapping population. In addition, we show that the level of expression of FaFAH1, with similarity to cytochrome p450 hydroxylases, significantly correlates with the content of γ-decalactone in the mapping population. The analysis of expression quantitative trait loci (eQTL) suggests that the product of this gene also has a regulatory role in the biosynthetic pathway of lactones.
Conclusions: Altogether, this study provides mechanistic information of how the production of γ-decalactone is naturally controlled in strawberry, and proposes enzymatic activities necessary for the formation of this VOC in plants.
Figures
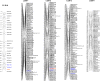
Comparison of pseudochromosome 3 (FV G-III) of the diploid Fragaria vesca with the four homoeologous linkage groups on the integrated ‘232’ × ‘1392’ linkage map (LGIII-1–LGIII-4) of the octoploid F. x ananassa. The γ-decalactone locus is highlighted in orange and linked SSR markers in blue. SSR and gene markers are highlighted in bold. Position of markers (in cM) is indicated on the left of the linkage groups. For simplicity, only the position of anchor SSR markers (in Mb) is stated on the left of the F. vesca group.
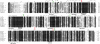
Comparison of the amino acid sequence of strawberry FaFAD1 with other plant fatty acid desaturases (Δ12-FADs) and hydroxilases (Δ12-FAH). Identical amino acid residues were indicated with black background. Dark and bright gray shade indicated 80% and 60% or more conservation among all the aligned sequences, respectively. The predicted trans-membrane domains (TM-helixes) and highly conserved His boxes are shown. The seven amino acid residues that differ between oleate desaturases and hydroxilases according to [45] (numbering based on the arabidopsis FAD2 sequence) are indicated in green when the amino acid residue is conserved between FaFAD1 and hydroxilases and red when not. Accession numbers for the sequences were as follows: Fragaria x ananassa FaFAD1 (KF887973), Nicotiana tabacum NtFAD (AAT72296), Olea europaea OeFAD2-1 (AAW63040), Davidia involucrate DiFAD2 (ABZ05022), Crepis alpina CaFAD2-2 (ABC00770), Arabidopsis thaliana AtFAD2 (AAA32782), Prunus persica PpFAD1B-6 (AGM53489), Ricinus comunis hydroxylase RcFAH12 (AAC49010), (MDP0000288297), Lesquerella fendleri bifunctional hydroxylase/desaturase LFAH12 (AAC32755).
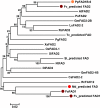
Phylogenetic tree showing protein sequence relationships among selected FAD2 members from different species. Bootstrap values (%) for 1000 replicates are indicated at the nodes. Position of the Fragaria, Malus and Prunus amino acid sequences is labeled in dark red, light red and orange, respectively. Accession numbers are shown in parenthesis: Prunus persica PpFAD1B-6 (AGM53489), Fragaria vesca Fv_predicted FAD (LOC101290788), Arabidopsis thaliana AtFAD2 (AAA32782), Persea americana PaFAD2 (AAL23676), Glycine max GmFAD2-2B (BAD89863), Linum usitatissimum LuFAD2-2 (ACF49507), Ricinus communis RcFAD2 (ABK59093), Theobroma cacao Tc_predicted FAD (EOY09487), Punica granatum PgFAD2 (AAO37754), Xanthoceras sorbifolia XsFAD2 (AGO32050), Olea europaea OeFAD2-1 (AAW63040), Sesamum indicum SiFAD2 (AAF80560), Solanum lycopersicum Sl_predicted FAD (XP_004228665), Nicotiana tabacum NtFAD (AAT72296), Davidia involucrate DiFAD2 (ABZ05022), Glycine max GmFAD2-1B (BAD89861), Crepis alpina CaFAD2-2 (ABC00770), Ricinus comunis RcFAH12 (AAC49010), Malus domestica Md_predicted FAD (MDP0000288297), Fragaria x ananassa FaFAD1 (KF887973) and Fragaria vesca Fv_predicted FAD1 (LOC101309231).
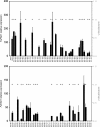
Comparison of expression of FaFAD1 in the 232 × 1392 mapping population by real time qRT-PCR with γ-decalactone content in fruits. FaFAD1 expression (black bars) is expressed relative to the average expression in the population and it is indicated on the Y-axis to the left. The production of γ-decalactone scored as presence/absence (gray dots) is indicated on the Y-axis to the right.
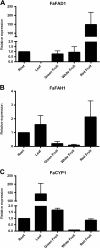
Expression profiles of candidate genes in different tissues and during fruit ripening determined by real-time qRT-PCR analysis. (A) Expression of FaFAD1, (B) expression of FaFAH1 and (C) expression of FaCYP1. Error bars indicate standard deviations from three biological replicates. Expression levels are expressed as a ratio relative to the root tissue.
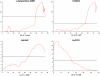
LOD profiles for γ-decalactone content and FaFAD1, FaFAH1 and FaCYP1 expression (shown in red) on linkage group III-2 for the former three and on LG III-1 for FaCYP1 (position is indicated in cM). Horizontal line marks the significant threshold for each QTL.
Similar articles
-
Chambers AH, Pillet J, Plotto A, Bai J, Whitaker VM, Folta KM. Chambers AH, et al. BMC Genomics. 2014 Apr 17;15:217. doi: 10.1186/1471-2164-15-217. BMC Genomics. 2014. PMID: 24742080 Free PMC article.
-
Acyl-CoA oxidase 1 is involved in γ-decalactone release from peach (Prunus persica) fruit.
Zhang L, Li H, Gao L, Qi Y, Fu W, Li X, Zhou X, Gao Q, Gao Z, Jia H. Zhang L, et al. Plant Cell Rep. 2017 Jun;36(6):829-842. doi: 10.1007/s00299-017-2113-4. Epub 2017 Feb 25. Plant Cell Rep. 2017. PMID: 28238071
-
Zorrilla-Fontanesi Y, Rambla JL, Cabeza A, Medina JJ, Sánchez-Sevilla JF, Valpuesta V, Botella MA, Granell A, Amaya I. Zorrilla-Fontanesi Y, et al. Plant Physiol. 2012 Jun;159(2):851-70. doi: 10.1104/pp.111.188318. Epub 2012 Apr 3. Plant Physiol. 2012. PMID: 22474217 Free PMC article.
-
Biotechnological production of γ-decalactone, a peach like aroma, by Yarrowia lipolytica.
Braga A, Belo I. Braga A, et al. World J Microbiol Biotechnol. 2016 Oct;32(10):169. doi: 10.1007/s11274-016-2116-2. Epub 2016 Aug 26. World J Microbiol Biotechnol. 2016. PMID: 27565779 Review.
-
Molecular Regulatory Mechanisms Affecting Fruit Aroma.
Lu H, Zhao H, Zhong T, Chen D, Wu Y, Xie Z. Lu H, et al. Foods. 2024 Jun 14;13(12):1870. doi: 10.3390/foods13121870. Foods. 2024. PMID: 38928811 Free PMC article. Review.
Cited by
-
Papaya (Carica papaya L.) Flavour Profiling.
Zhou Z, Ford R, Bar I, Kanchana-Udomkan C. Zhou Z, et al. Genes (Basel). 2021 Sep 15;12(9):1416. doi: 10.3390/genes12091416. Genes (Basel). 2021. PMID: 34573398 Free PMC article. Review.
-
Geng J, Cao Q, Jiang S, Huangfu J, Wang W, Niu Z. Geng J, et al. Foods. 2024 Apr 23;13(9):1290. doi: 10.3390/foods13091290. Foods. 2024. PMID: 38731661 Free PMC article.
-
Sensory sacrifices when we mass-produce mass produce.
Folta KM, Klee HJ. Folta KM, et al. Hortic Res. 2016 Jul 13;3:16032. doi: 10.1038/hortres.2016.32. eCollection 2016. Hortic Res. 2016. PMID: 27602229 Free PMC article. Review.
-
Study on VOCs of Fishmeal during Storage Based on HS-SPME-GC-MS.
Geng J, Nie K, Wang W, Jiang S, Niu Z. Geng J, et al. ACS Omega. 2024 Jul 17;9(30):32817-32827. doi: 10.1021/acsomega.4c03323. eCollection 2024 Jul 30. ACS Omega. 2024. PMID: 39100347 Free PMC article.
-
Muñoz P, Castillejo C, Gómez JA, Miranda L, Lesemann S, Olbricht K, Petit A, Chartier P, Haugeneder A, Trinkl J, Mazzoni L, Masny A, Zurawicz E, Ziegler FMR, Usadel B, Schwab W, Denoyes B, Mezzetti B, Osorio S, Sánchez-Sevilla JF, Amaya I. Muñoz P, et al. Hortic Res. 2023 Jan 19;10(3):uhad006. doi: 10.1093/hr/uhad006. eCollection 2023 Mar. Hortic Res. 2023. PMID: 36938573 Free PMC article.
References
-
- Pérez A, Sanz A. In: Handbook of Fruit and Vegetable Flavors. Hui HY, editor. Hoboken, New Jersey: John Wiley & Sons, Inc; 2010. Strawberry flavor; pp. 437–455.
-
- Latrasse A. In: Volatile Compounds in Fruits and Beverages. Maarse H, editor. New York: Marcek Dekker, Inc; 1991. Fruits III; pp. 329–387.
-
- Jetti RR, Yang E, Kurnianta A, Finn C, Qian MC. Quantification of selected aroma-active compounds in strawberries by headspace solid-phase microextraction gas chromatography and correlation with sensory descriptive analysis. J Food Sci. 2007;15:S487–S496. doi: 10.1111/j.1750-3841.2007.00445.x. - DOI - PubMed
Publication types
MeSH terms
Substances
LinkOut - more resources
Full Text Sources
Other Literature Sources
Research Materials