Validation of a fluorescence-based screening concept to identify ribosome assembly defects in Escherichia coli - PubMed
Validation of a fluorescence-based screening concept to identify ribosome assembly defects in Escherichia coli
Rainer Nikolay et al. Nucleic Acids Res. 2014 Jul.
Abstract
While the structure of mature ribosomes is analyzed in atomic detail considerably less is known about their assembly process in living cells. This is mainly due to technical and conceptual hurdles. To analyze ribosome assembly in vivo, we designed and engineered an Escherichiacoli strain--using chromosomal gene knock-in techniques--that harbors large and small ribosomal subunits labeled with the fluorescent proteins EGFP and mCherry, respectively. A thorough characterization of this reporter strain revealed that its growth properties and translation apparatus were wild-type like. Alterations in the ratio of EGFP over mCherry fluorescence are supposed to indicate ribosome assembly defects. To provide proof of principle, subunit specific assembly defects were provoked and could be identified by both manual and fully automated fluorometric in vivo assays. This is to our knowledge the first methodology that directly detects ribosome assembly defects in vivo in a high-throughput compatible format. Screening of knock-out collections and small molecule libraries will allow identification of new ribosome assembly factors and possible inhibitors.
© The Author(s) 2014. Published by Oxford University Press on behalf of Nucleic Acids Research.
Figures
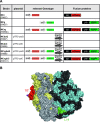
Overview of constructed strains and 70S ribosome structure. (A) Given are the names of the constructed strains as used in this study (lab nomenclature in brackets), relevant genotype and fluorescent fusion proteins produced. pTRC-rpsQ, pTRC-rplC: complementation plasmids with copies of the chromosomally deleted genes. Endogenous genes are shown as gray arrows, genes encoding FPs as colored boxes. Genes to be deleted were replaced by kanamycin resistance cassettes (KanR). mCherry gene and protein portions are shown in red, EGFP accordingly in green. (B) Surface representation of an E. coli 70S ribosome crystal structure. The 16S rRNA is colored in light gray proteins of the small subunit in yellow. 23S and 5S rRNA are shown in dark gray, proteins of the large subunit in cyan. S2 is highlighted in red, L19 in green. Their surface exposed C-termini are shown in purple. The figure was generated with pymol, based on PDB files 3R8S and 4GD1 (28).
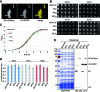
Physiological and biochemical characterization. (A) Fluorescence micrographs of MCrg cells. Cells were grown in LB medium at 37°C to mid-logarithmic phase and spotted onto a thin agarose pad. The agarose pad was sealed with a cover slip and subjected to microscopic analysis. Scale bar: 5 μm. (B) Growth comparison on solid medium: Cells of the indicated strains were spotted onto LB agar in a serial dilution and incubated at the given temperatures. For details see Materials and methods. (C) Growth comparison in liquid medium: Cells of the indicated strains were grown in LB medium at 37°C for 6 h. OD600 values were determined every hour and plotted against time. (D) Cells as indicated were grown at 20, 37 and 42°C to stationary phase. Growth rates were calculated and normalized values are given for each strain at each incubation temperature. N = 3. (E) Ribosomes from the indicated strains were isolated by sucrose cushion centrifugation and subjected to SDS-PAGE and western blot (WB) analysis. For immunodetection S2 and L19 specific antisera were used. Asterisks denote typical product of chromophore hydrolysis of ds red derivatives (26).
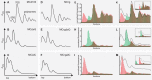
Polysome analysis and fluorescence detection of sucrose fractions. Cells were grown in M9 medium at 37°C to OD600 = 0.4 and harvested. Lysates were subjected to sucrose gradient centrifugation. Centrifugates were analyzed by A254 detection and fractionated. Polysome profiles derived from (A) MC4100, (B) MCΔsQ, (C) MCΔlC, (D) MCrg, (E) MCrgΔsQ, (F) MCrgΔlC. Sucrose gradient fractions of samples D–F were analyzed for EGFP- and mCherry-specific fluorescence and normalized results are given in bar charts for (G) MCrg, (H), MCrgΔsQ and (I) MCrgΔlC. Superposition of A254 profiles and corresponding fluorescence bar charts: (K) MCrg, (L) MCrgΔsQ, (M) MCrgΔlC. The inserts show fluorescence analysis of all available fractions from each sucrose gradient run. Red bars: normalized mCherry fluorescence; Green bars: normalized EGFP fluorescence. Fluorescence was normalized to 70S peak (‘monosome’) where subunits are supposed to be present in 1:1 ratio. However, the 70S peak contains the tail of the 50S peak, therefore normalization leads to underestimation of the EGFP signal intensity.
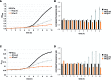
Growth in flasks and manual fluorescence analysis of reporter strains. The strains MC4100, MCΔsQ, MCΔlC, MCrg, MCrgΔsQ and MCrgΔlC were cultured in Erlenmeyer flasks at 37°C in M9 medium for 9 h. In 1-h intervals samples were taken. (A) OD600 values were determined and (B) EGFP and mCherry fluorescence emission were detected and ratios were calculated for MCrg, MCrgΔsQ and MCrgΔlC. Fluorescence ratios of MCrg were normalized to 1. One exemplary growth curve is given and fluorescence ratios are means from three independent experiments; error bars show s.d. Growth in 384-well plates and fully automated fluorescence analysis of reporter strains. Aliquots of MC4100, MCΔsQ, MCΔlC, MCrg, MCrgΔsQ and MCrgΔlC cultures were transferred into 384- well plates in quadruplicates. Cells were grown in M9 medium at 37°C for 10 h. Samples were taken in 1-h intervals. (C) A650 values were determined and (D) EGFP and mCherry fluorescence emission were detected and ratios were calculated for MCrg, MCrgΔsQ and MCrgΔlC. Fluorescence ratios of MCrg were normalized to 1. A representative growth curve is shown and fluorescence ratios are mean values; n = 4, error bars show s.d.
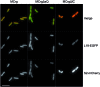
Fluorescence microscopic comparison of MCrg, MCrgΔsQ and MCrgΔlC. MCrg and MCrgΔsQ cells were grown in M9 medium to OD600 = 0.4, whereas MCrgΔlC cells were grown to OD600 = 0.12. Representative fluorescence images were taken. Lower row: red channel (S2-mCherry); middle row: green channel (L19-EGFP); the upper row is an overlay of both channels (merge). Scale bar: 5 μm.
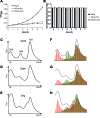
Testing MCrg with inhibitors of translation. Whole-cell analyses: MCrg cells were cultured in Erlenmeyer flasks at 25°C in M9 medium for 7 h in the absence and presence of antibiotics, as indicated. Samples were taken every hour. (A) OD600 values were determined and (B) EGFP and mCherry fluorescence emission were detected and ratios were calculated. Fluorescence ratios of MCrg were normalized to 1. Exemplary growth curves are given and fluorescence ratios are means from three independent experiments; error bars show s.d. Analyses of isolated ribosomal particles: Sucrose density gradient (10–25%) centrifugation profiles from (C) control cells with no antibiotic (none), (D) chloramphenicol (Cam) and (E) erythromycin (Ery) treated cells. Sucrose gradient fractions from (C), (D) and (E) were analyzed for fluorescence by a microplate reader. A254 profiles and fluorescence bar charts were superimposed for (F) control cells with no antibiotic (none), (G) chloramphenicol (Cam) and (H) erythromycin (Ery) treated cells. Cells in presence and absence of antibiotics were cultured in M9 medium at 25°C for 3 h before subsequent polysome analysis. Red bars: normalized mCherry fluorescence; Green bars: normalized EGFP fluorescence. Fluorescence was normalized to the first polysome peak (‘disome’) where subunits are present in 1:1 ratio.
Similar articles
-
Fluorescence-based monitoring of ribosome assembly landscapes.
Nikolay R, Schloemer R, Mueller S, Deuerling E. Nikolay R, et al. BMC Mol Biol. 2015 Feb 25;16:3. doi: 10.1186/s12867-015-0031-y. BMC Mol Biol. 2015. PMID: 25884162 Free PMC article.
-
Quantitative proteomic analysis of ribosome assembly and turnover in vivo.
Sykes MT, Shajani Z, Sperling E, Beck AH, Williamson JR. Sykes MT, et al. J Mol Biol. 2010 Oct 29;403(3):331-45. doi: 10.1016/j.jmb.2010.08.005. Epub 2010 Aug 13. J Mol Biol. 2010. PMID: 20709079 Free PMC article.
-
Ederth J, Mandava CS, Dasgupta S, Sanyal S. Ederth J, et al. Nucleic Acids Res. 2009 Feb;37(2):e15. doi: 10.1093/nar/gkn992. Epub 2008 Dec 11. Nucleic Acids Res. 2009. PMID: 19074194 Free PMC article.
-
[Ribosome: lessons of a molecular factory construction].
Sergeeva OV, Sergiev PV, Bogdanov AA, Dontsova OA. Sergeeva OV, et al. Mol Biol (Mosk). 2014 Jul-Aug;48(4):543-60. Mol Biol (Mosk). 2014. PMID: 25842841 Review. Russian.
-
RNA folding and ribosome assembly.
Woodson SA. Woodson SA. Curr Opin Chem Biol. 2008 Dec;12(6):667-73. doi: 10.1016/j.cbpa.2008.09.024. Epub 2008 Oct 18. Curr Opin Chem Biol. 2008. PMID: 18935976 Free PMC article. Review.
Cited by
-
Ribosome assembly defects subvert initiation Factor3 mediated scrutiny of bona fide start signal.
Sharma H, Anand B. Sharma H, et al. Nucleic Acids Res. 2019 Dec 2;47(21):11368-11386. doi: 10.1093/nar/gkz825. Nucleic Acids Res. 2019. PMID: 31586395 Free PMC article.
-
Techniques for Screening Translation Inhibitors.
Osterman IA, Bogdanov AA, Dontsova OA, Sergiev PV. Osterman IA, et al. Antibiotics (Basel). 2016 Jun 24;5(3):22. doi: 10.3390/antibiotics5030022. Antibiotics (Basel). 2016. PMID: 27348012 Free PMC article. Review.
-
Stukenberg D, Hoff J, Faber A, Becker A. Stukenberg D, et al. Commun Biol. 2022 Mar 25;5(1):265. doi: 10.1038/s42003-022-03150-0. Commun Biol. 2022. PMID: 35338236 Free PMC article.
-
Fluorescence-based monitoring of ribosome assembly landscapes.
Nikolay R, Schloemer R, Mueller S, Deuerling E. Nikolay R, et al. BMC Mol Biol. 2015 Feb 25;16:3. doi: 10.1186/s12867-015-0031-y. BMC Mol Biol. 2015. PMID: 25884162 Free PMC article.
-
Gawin A, Peebo K, Hans S, Ertesvåg H, Irla M, Neubauer P, Brautaset T. Gawin A, et al. Microb Cell Fact. 2019 May 7;18(1):80. doi: 10.1186/s12934-019-1128-7. Microb Cell Fact. 2019. PMID: 31064376 Free PMC article.
References
Publication types
MeSH terms
Substances
LinkOut - more resources
Full Text Sources
Other Literature Sources
Molecular Biology Databases