Metformin impairs mitochondrial function in skeletal muscle of both lean and diabetic rats in a dose-dependent manner - PubMed
- ️Wed Jan 01 2014
Metformin impairs mitochondrial function in skeletal muscle of both lean and diabetic rats in a dose-dependent manner
Bart Wessels et al. PLoS One. 2014.
Abstract
Metformin is a widely prescribed drug for the treatment of type 2 diabetes. Previous studies have demonstrated in vitro that metformin specifically inhibits Complex I of the mitochondrial respiratory chain. This seems contraindicative since muscle mitochondrial dysfunction has been linked to the pathogenesis of type 2 diabetes. However, its significance for in vivo skeletal muscle mitochondrial function has yet to be elucidated. The aim of this study was to assess the effects of metformin on in vivo and ex vivo skeletal muscle mitochondrial function in a rat model of diabetes. Healthy (fa/+) and diabetic (fa/fa) Zucker diabetic fatty rats were treated by oral gavage with metformin dissolved in water (30, 100 or 300 mg/kg bodyweight/day) or water as a control for 2 weeks. After 2 weeks of treatment, muscle oxidative capacity was assessed in vivo using 31P magnetic resonance spectroscopy and ex vivo by measuring oxygen consumption in isolated mitochondria using high-resolution respirometry. Two weeks of treatment with metformin impaired in vivo muscle oxidative capacity in a dose-dependent manner, both in healthy and diabetic rats. Whereas a dosage of 30 mg/kg/day had no significant effect, in vivo oxidative capacity was 21% and 48% lower after metformin treatment at 100 and 300 mg/kg/day, respectively, independent of genotype. High-resolution respirometry measurements demonstrated a similar dose-dependent effect of metformin on ex vivo mitochondrial function. In conclusion, metformin compromises in vivo and ex vivo muscle oxidative capacity in Zucker diabetic fatty rats in a dose-dependent manner.
Conflict of interest statement
Competing Interests: The authors have declared that no competing interests exist.
Figures
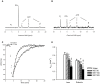
Representative examples of 31P MR spectra obtained during rest with 32 averages (A) and at the end of the electrical-stimulation protocol with 4 averages (B). (C) Representative examples of relative PCr concentrations during rest, muscle stimulation and recovery (time resolution = 20 s) for a water-treated diabetic rat (open symbols) and a diabetic rat treated with metformin at 300 mg/kg body weight/day (filled symbols). PCr concentrations are expressed as a percentage of the resting PCr concentration. Mono-exponential functions (dark lines) were fit to the recovery data and the PCr recovery rate constants were 0.63 and 0.21 min-1 for the water-treated and metformin-treated animal, respectively. (D) Rate constants of PCr recovery, k PCr, after electrical stimulation in TA muscle of lean and diabetic rats treated with water or 30, 100 or 300 mg/kg body weight/day metformin (MET30, MET100 and MET300 respectively). Data is represented as mean ± SD (n = 6 per group). k PCr was significantly lower in diabetic rats compared with lean rats, independent of treatment regimen (ANOVA: P<0.001). In addition, treatment had a significant effect on k PCr, independent of genotype, and a pairwise analysis of differences is provided by Bonferroni-corrected post-hoc tests: * P<0.001 when compared with water-treated animals, † P<0.001 when compared with MET30-treated animals, ‡ P<0.001 when compared with MET100-treated animals.
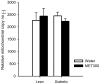
Data is represented as mean ± SD (n = 6 per group).
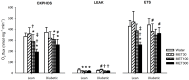
Respiratory capacity was determined in the OXPHOS state, when mitochondrial respiration is coupled to ATP synthesis; the LEAK-state, when the system is limited by ADP; and the ETS state, after uncoupling of the ETS from ATP synthesis. Data is represented as mean ± SD (n = 6 per group). For the OXPHOS state, the interaction between genotype and treatment was borderline significant and for the LEAK and ETS state, the interaction between genotype and treatment was significant. A pairwise analysis of differences is provided by Bonferroni-corrected two-sided unpaired t-tests: * P<0.05 when compared with water-treated animals of the same genotype, † P<0.05 when compared with MET30-treated animals of the same genotype, ‡ P<0.05 when compared with MET100-treated animals of the same genotype, # P<0.05 when compared with lean animals of the same treatment regimen.
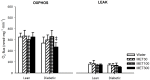
Respiratory capacity was determined in the OXPHOS state, when mitochondrial respiration is coupled to ATP synthesis; and the LEAK-state, when the system is limited by ADP. Data is represented as mean ± SD (n = 6 per group). For the OXPHOS state, the interaction between genotype and treatment was significant and a pairwise analysis of differences is provided by Bonferroni-corrected two-sided unpaired t-tests: ‡ P<0.05 when compared with MET100-treated animals of the same genotype.
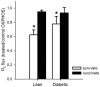
Respiratory capacity was determined in the OXPHOS state, when mitochondrial respiration is coupled to ATP synthesis, fueled with either pyruvate plus malate (Complex I respiration) or succinate plus rotenone (Complex II respiration). Data is represented as mean ± SD (n = 6 per group). Incubation with metformin significantly lowered OXPHOS respiration fueled with pyruvate plus malate, independent of genotype (ANOVA: * P<0.001). Metformin did not affect Complex II respiration.
Similar articles
-
Pioglitazone treatment restores in vivo muscle oxidative capacity in a rat model of diabetes.
Wessels B, Ciapaite J, van den Broek NM, Houten SM, Nicolay K, Prompers JJ. Wessels B, et al. Diabetes Obes Metab. 2015 Jan;17(1):52-60. doi: 10.1111/dom.12388. Epub 2014 Oct 6. Diabetes Obes Metab. 2015. PMID: 25200673
-
Kane DA, Anderson EJ, Price JW 3rd, Woodlief TL, Lin CT, Bikman BT, Cortright RN, Neufer PD. Kane DA, et al. Free Radic Biol Med. 2010 Sep 15;49(6):1082-7. doi: 10.1016/j.freeradbiomed.2010.06.022. Epub 2010 Jun 28. Free Radic Biol Med. 2010. PMID: 20600832 Free PMC article.
-
De Feyter HM, Lenaers E, Houten SM, Schrauwen P, Hesselink MK, Wanders RJ, Nicolay K, Prompers JJ. De Feyter HM, et al. FASEB J. 2008 Nov;22(11):3947-55. doi: 10.1096/fj.08-112318. Epub 2008 Jul 24. FASEB J. 2008. PMID: 18653763
-
Type 2 diabetes mellitus and skeletal muscle metabolic function.
Phielix E, Mensink M. Phielix E, et al. Physiol Behav. 2008 May 23;94(2):252-8. doi: 10.1016/j.physbeh.2008.01.020. Epub 2008 Jan 31. Physiol Behav. 2008. PMID: 18342897 Review.
-
Chanséaume E, Morio B. Chanséaume E, et al. Int J Mol Sci. 2009 Jan;10(1):306-324. doi: 10.3390/ijms10010306. Epub 2009 Jan 13. Int J Mol Sci. 2009. PMID: 19333447 Free PMC article. Review.
Cited by
-
PFKFB3-mediated glycolysis rescues myopathic outcomes in the ischemic limb.
Ryan TE, Schmidt CA, Tarpey MD, Amorese AJ, Yamaguchi DJ, Goldberg EJ, Iñigo MM, Karnekar R, O'Rourke A, Ervasti JM, Brophy P, Green TD, Neufer PD, Fisher-Wellman K, Spangenburg EE, McClung JM. Ryan TE, et al. JCI Insight. 2020 Sep 17;5(18):e139628. doi: 10.1172/jci.insight.139628. JCI Insight. 2020. PMID: 32841216 Free PMC article.
-
Differential metformin dose-dependent effects on cognition in rats: role of Akt.
Mostafa DK, Ismail CA, Ghareeb DA. Mostafa DK, et al. Psychopharmacology (Berl). 2016 Jul;233(13):2513-24. doi: 10.1007/s00213-016-4301-2. Epub 2016 Apr 25. Psychopharmacology (Berl). 2016. PMID: 27113224
-
Moreno-Cabañas A, Morales-Palomo F, Alvarez-Jimenez L, Ortega JF, Mora-Rodriguez R. Moreno-Cabañas A, et al. Obesity (Silver Spring). 2022 Jun;30(6):1219-1230. doi: 10.1002/oby.23410. Epub 2022 May 17. Obesity (Silver Spring). 2022. PMID: 35578807 Free PMC article.
-
Metformin delays neurological symptom onset in a mouse model of neuronal complex I deficiency.
Peralta S, Pinto M, Arguello T, Garcia S, Diaz F, Moraes CT. Peralta S, et al. JCI Insight. 2020 Nov 5;5(21):e141183. doi: 10.1172/jci.insight.141183. JCI Insight. 2020. PMID: 33148885 Free PMC article.
-
Erickson ML, Allen JM, Beavers DP, Collins LM, Davidson KW, Erickson KI, Esser KA, Hesselink MKC, Moreau KL, Laber EB, Peterson CA, Peterson CM, Reusch JE, Thyfault JP, Youngstedt SD, Zierath JR, Goodpaster BH, LeBrasseur NK, Buford TW, Sparks LM. Erickson ML, et al. Geroscience. 2023 Feb;45(1):569-589. doi: 10.1007/s11357-022-00668-3. Epub 2022 Oct 15. Geroscience. 2023. PMID: 36242693 Free PMC article. Review.
References
-
- Argaud D, Roth H, Wiernsperger N, Leverve XM (1993) Metformin decreases gluconeogenesis by enhancing the pyruvate kinase flux in isolated rat hepatocytes. Eur J Biochem 213: 1341–1348. - PubMed
-
- Brunmair B, Staniek K, Gras F, Scharf N, Althaym A, et al. (2004) Thiazolidinediones, like metformin, inhibit respiratory complex I: a common mechanism contributing to their antidiabetic actions? Diabetes 53: 1052–1059. - PubMed
-
- Carvalho C, Correia S, Santos MS, Seica R, Oliveira CR, et al. (2008) Metformin promotes isolated rat liver mitochondria impairment. Mol Cell Biochem 308: 75–83. - PubMed
Publication types
MeSH terms
Substances
Grants and funding
B.W. and J.J.P. are supported by a VIDI grant (project number 700.58.421) from the Netherlands Organisation for Scientific Research (NWO). J.C. is supported by the NWO-funded Groningen Systems Biology Center for Energy Metabolism and Aging. The funders had no role in study design, data collection and analysis, decision to publish, or preparation of the manuscript.
LinkOut - more resources
Full Text Sources
Other Literature Sources
Medical