Conjugated bile acid-activated S1P receptor 2 is a key regulator of sphingosine kinase 2 and hepatic gene expression - PubMed
. 2015 Apr;61(4):1216-26.
doi: 10.1002/hep.27592. Epub 2015 Mar 9.
Kazuaki Takabe, Runping Liu, Kesong Peng, Xiang Wang, Yun Wang, Nitai C Hait, Xuan Wang, Jeremy C Allegood, Akimitsu Yamada, Tomoyoshi Aoyagi, Jie Liang, William M Pandak, Sarah Spiegel, Phillip B Hylemon, Huiping Zhou
Affiliations
- PMID: 25363242
- PMCID: PMC4376566
- DOI: 10.1002/hep.27592
Conjugated bile acid-activated S1P receptor 2 is a key regulator of sphingosine kinase 2 and hepatic gene expression
Masayuki Nagahashi et al. Hepatology. 2015 Apr.
Abstract
Bile acids are important hormones during the feed/fast cycle, allowing the liver to coordinately regulate nutrient metabolism. How they accomplish this has not been fully elucidated. Conjugated bile acids activate both the ERK1/2 and AKT signaling pathways via sphingosine 1-phosphate receptor 2 (S1PR2) in rodent hepatocytes and in vivo. Here, we report that feeding mice a high-fat diet, infusion of taurocholate into the chronic bile fistula rat, or overexpression of the gene encoding S1PR2 in mouse hepatocytes significantly upregulated hepatic sphingosine kinase 2 (SphK2) but not SphK1. Key genes encoding nuclear receptors/enzymes involved in nutrient metabolism were significantly downregulated in livers of S1PR2(-/-) and SphK2(-/-) mice. In contrast, overexpression of the gene encoding S1PR2 in primary mouse hepatocytes differentially increased SphK2, but not SphK1, and mRNA levels of key genes involved in nutrient metabolism. Nuclear levels of sphingosine-1-phosphate, an endogenous inhibitor of histone deacetylases 1 and 2, as well as the acetylation of histones H3K9, H4K5, and H2BK12 were significantly decreased in hepatocytes prepared from S1PR2(-/-) and SphK2(-/-) mice.
Conclusion: Both S1PR2(-/-) and SphK2(-/-) mice rapidly developed fatty livers on a high-fat diet, suggesting the importance of conjugated bile acids, S1PR2, and SphK2 in regulating hepatic lipid metabolism.
© 2014 by the American Association for the Study of Liver Diseases.
Conflict of interest statement
Competing Financial interest: The authors declare no competing financial interest.
Figures
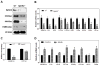
(A) Nuclear extracts of primary hepatocytes isolated from Wild type (WT) and SphK2−/− mice were prepared as described in “Methods”. The protein levels of SphK2, H3K9ac, H4K5ac, H2BK12ac and total H3 were determined by Western blot analysis. Representative images are shown. (B) Total RNA was isolated from the livers of WT (solid bar) or SphK2−/− mice (checkered bar) (male, 20-week old). The mRNA levels of key genes involved in lipid metabolism were determined using real time RT-PCR and normalized using GAPDH. Abbs. SREBP-1c: sterol regulatory element-binding protein-1c; FAS: fatty acid synthase; LDLR: low-density lipoprotein receptor; CYP27A1: sterol 27-hydroxylase; CYP7A1: cholesterol 7 α-hydroxylase; BSEP: bile salt export pump; FXR: farnesoid X receptor; BECN1: autophagy-related gene (Atg) 6; PPARγ: peroxisome proliferator-activated receptor γ. *P<0.05, **P<0.01, ***P<0.01, statistical significance relative to WT mice, n=5. (C) Effect of SphK2 on association of acetylated H3K9 in SREBP1c and CYP7A1 chromatins. Mouse primary hepatocytes were isolated from wild type (WT) and SphK2−/− mice. ChIP assay and real-time PCR were used to quantify the DNA amount of SREBP1c and CYP7A1 associated with H3K9ac as described in “Methods”. Results were expressed as relative abundance of chromatin DNA associated with H3K9ac. Values are mean ± S.E. **P<0.01, statistical significance relative to WT, n=3. (D) The wild type mouse primary hepatocytes (solid bar) were treated with a chemical inhibitor of HDAC, SAHA (1 μM) for 8h (checkered bar). The total RNA was isolated. The mRNA levels of key genes involved in lipid metabolism were determined using real time PCR and normalized using β-actin. CPT-1α: Carnitine palmitoyltransferase I α; PCG1α: Peroxisome proliferator-activated receptor gamma coactivator 1-alpha; ACC1: Acetyl-CoA carboxylase 1.
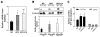
Wild type (WT) and SphK2−/− (KO) mice (male, 20-week old) were fed normal chow diet (ND; solid bar), high fat diet (HFD; checkered bar), or HFD containing 1% cholic acid (HFD+CA; shaded bar) for two weeks. (A) The mRNA levels of SphK2 in the liver of WT mice were determined by real time RT-PCR and normalized using GAPDH as internal control. (B) The protein expression levels of SphK2 in the liver of WT and SphK2−/− mice were determined by Western Blot analysis and normalized using β-tubulin as loading control. (C) The enzyme activities of SphK2 were measured using [γ32P]-ATP. *P<0.05, **P<0.01, statistical significance relative to control group.
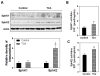
Bile fistulas were placed in rats and the bile was drained for 48 hrs. TCA was infused intraduodenally at a rate of 1.05 ml/h/100 g rat and a concentration of 36 μmol/h/100 g rat for 4h (checkered bar). Animals were harvested and the liver pieces from each animal were isolated and snap-frozen. The protein levels of SphK1 and SphK2 were detected by Western blot analysis using antibodies against SphK1 and SphK2 as described in “Methods”. (A) Representative images of immunoblots for SphK1, SphK2 and actin are shown. Relative densities of SphK1 and SphK2 were determined by scanning laser density spectrometry and actin was used as a loading control. (B and C) The specific enzyme activities of SphK1 and SphK2 were determined using [γ32P]-ATP and sphingosine as described in “Methods”. *p<0.05, statistical significance relative to control group, n=5.
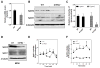
(A) Total RNA was isolated from the liver of Wild type (WT) and S1PR2−/− mice. The mRNA levels of SphK2 were determined using real-time RT-PCR. (B) The total liver protein lysates of WT and S1PR2−/− mice were prepared and protein levels of SphK1 and SphK2 were determined by Western blot analysis. Representative images are shown. (C) The relative densities of SphK1 (solid bar) and SphK2 (checkered bar) were determined using β-tubulin as a loading control. (D) Primary mouse hepatocytes were isolated from Wild type (WT) and S1PR2−/− mice. The protein levels of SphK1 and SphK2 were determined by Western Blot analysis and the representative images are shown. β-tubulin was used as a loading control. (E and F) Primary mouse hepatocytes isolated from WT (closed circle) and S1PR2−/− (closed diamond) mice were treated with TCA (100 μM) for 0–30 min). The enzyme activities of SphK1 and SphK2 were measured using [γ32P]-ATP and sphingosine.
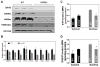
(A) Nuclear extracts of primary hepatocytes isolated from Wild type (WT) and S1PR2−/− mice were prepared as described in “Methods”. The protein levels of SphK2, H3K9ac, H4K5ac, H2BK12ac and total H3 were determined by Western blot analysis. Representative images are shown. (B) Total RNA was isolated from the livers of WT (solid bar) or S1PR2−/− (checkered bar) mice (male, 20-week old). The mRNA levels of key genes involved in lipid metabolism were determined using real time RT-PCR and normalized using β-actin. Abbs: SREBP-1c: sterol regulatory element-binding protein-1c; FAS: fatty acid synthase; LDLR: low-density lipoprotein receptor; CYP27A1: sterol 27-hydroxylase; CYP7A1: cholesterol 7 α-hydroxylase; BSEP: bile salt export pump; FXR: farnesoid X receptor. BECN1: autophagy-related gene (Atg) 6; PPARγ: peroxisome proliferator-activated receptor γ. *P<0.05, **P<0.01, ***P<0.01, statistical significance relative to WT mice, n=5–8. (C and D) The S1P and DHS1P levels in the cytosol and nucleus of mouse primary hepatocytes isolated from WT and S1PR2−/− mice were measured using mass spectrometry as described in “Methods”. *P<0.05, statistical significance relative to WT.
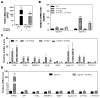
The mouse primary hepatocytes isolated from wild type (WT) or S1PR2−/− mice were transfected with a control vector or pcDNA3-mS1PR2 as described in “Methods”. The total cellular RNA was isolated after 48h. The mRNA levels of specific target genes were determined using real-time RT-PCR and normalized using GAPDH as an internal control. (A) The mRNA levels of S1PR2; (B) The mRNA levels of SphK1 and SphK2; (C) The mRNA levels of key genes involved in lipid metabolism. *P<0.05, **P<0.01, ***P<0.001, statistical significance relative to control vector transfected cells. (D) Effect of overexpression of the gene encoding S1PR2 on gene regulation in SphK2−/− mouse primary hepatocytes. The mouse primary hepatocytes isolated from SphK2−/− mice were transfected with a control vector or pcDNA3-mS1PR2 as described above. The total cellular RNA was isolated after 48h. The mRNA levels of specific target genes were determined using real-time RT-PCR and normalized using GAPDH as an internal control. The mRNA levels of key genes involved in lipid metabolism. *P<0.05, **P<0.01, ***P<0.001, statistical significance relative to control vector transfected cells.
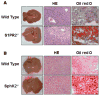
Wild type (WT), S1PR2−/− and SphK2−/− mice (male, 20-week old) were fed HFD for two weeks. The liver sections were stained using Oil Red O or HE. The images were taken with an Olympus microscope equipped with image recorder using a 400x lens. Representative images of livers, HE staining and Oil Red O staining are shown. (A) S1PR2−/− mice; (B) SphK2−/− mice.
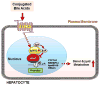
CBA returning from the intestines following a meal activates S1PR2. Activation of this GPCR then activates nuclear SphK2 via cell signaling pathways increasing the levels of S1P in the nucleus. Nuclear S1P inhibits specific histone deacetylases (HDACs) causing an increase in acetylation of histones and up-regulation of genes encoding nuclear receptors and enzymes involved in nutrient metabolism. This CBA-activated nutrient signaling pathway is hypothesized to allow the liver to be more efficient in metabolizing a bolus of incoming nutrients allowing the liver to maintain “nutrient homeostasis”.
Comment in
-
Chiang JY. Chiang JY. Hepatology. 2015 Apr;61(4):1118-20. doi: 10.1002/hep.27616. Epub 2015 Mar 10. Hepatology. 2015. PMID: 25418695 Free PMC article. No abstract available.
-
Hylemon P, Nagahashi M, Takabe K, Zhou H. Hylemon P, et al. Hepatology. 2016 May;63(5):1740-1. doi: 10.1002/hep.27961. Epub 2015 Jul 31. Hepatology. 2016. PMID: 26121965 Free PMC article. No abstract available.
-
Bile acids and nonalcoholic fatty liver disease: An intriguing relationship.
Carulli L, Gabbi C, Bertolotti M. Carulli L, et al. Hepatology. 2016 May;63(5):1739-40. doi: 10.1002/hep.27963. Epub 2015 Jul 31. Hepatology. 2016. PMID: 26122405 No abstract available.
Similar articles
-
Conjugated bile acids activate the sphingosine-1-phosphate receptor 2 in primary rodent hepatocytes.
Studer E, Zhou X, Zhao R, Wang Y, Takabe K, Nagahashi M, Pandak WM, Dent P, Spiegel S, Shi R, Xu W, Liu X, Bohdan P, Zhang L, Zhou H, Hylemon PB. Studer E, et al. Hepatology. 2012 Jan;55(1):267-76. doi: 10.1002/hep.24681. Epub 2011 Nov 30. Hepatology. 2012. PMID: 21932398 Free PMC article.
-
Karimian G, Buist-Homan M, Schmidt M, Tietge UJ, de Boer JF, Klappe K, Kok JW, Combettes L, Tordjmann T, Faber KN, Moshage H. Karimian G, et al. Biochim Biophys Acta. 2013 Dec;1832(12):1922-9. doi: 10.1016/j.bbadis.2013.06.011. Epub 2013 Jun 28. Biochim Biophys Acta. 2013. PMID: 23816565
-
Bile acids and sphingosine-1-phosphate receptor 2 in hepatic lipid metabolism.
Kwong E, Li Y, Hylemon PB, Zhou H. Kwong E, et al. Acta Pharm Sin B. 2015 Mar;5(2):151-7. doi: 10.1016/j.apsb.2014.12.009. Epub 2015 Feb 9. Acta Pharm Sin B. 2015. PMID: 26579441 Free PMC article. Review.
-
Wang Y, Aoki H, Yang J, Peng K, Liu R, Li X, Qiang X, Sun L, Gurley EC, Lai G, Zhang L, Liang G, Nagahashi M, Takabe K, Pandak WM, Hylemon PB, Zhou H. Wang Y, et al. Hepatology. 2017 Jun;65(6):2005-2018. doi: 10.1002/hep.29076. Epub 2017 Apr 28. Hepatology. 2017. PMID: 28120434 Free PMC article.
-
Bile acids are nutrient signaling hormones.
Zhou H, Hylemon PB. Zhou H, et al. Steroids. 2014 Aug;86:62-8. doi: 10.1016/j.steroids.2014.04.016. Epub 2014 May 10. Steroids. 2014. PMID: 24819989 Free PMC article. Review.
Cited by
-
Hirose Y, Nagahashi M, Katsuta E, Yuza K, Miura K, Sakata J, Kobayashi T, Ichikawa H, Shimada Y, Kameyama H, McDonald KA, Takabe K, Wakai T. Hirose Y, et al. Sci Rep. 2018 Jul 17;8(1):10814. doi: 10.1038/s41598-018-29144-9. Sci Rep. 2018. PMID: 30018456 Free PMC article.
-
Okano M, Oshi M, Butash A, Okano I, Saito K, Kawaguchi T, Nagahashi M, Kono K, Ohtake T, Takabe K. Okano M, et al. J Mammary Gland Biol Neoplasia. 2020 Mar;25(1):27-36. doi: 10.1007/s10911-020-09442-7. Epub 2020 Feb 27. J Mammary Gland Biol Neoplasia. 2020. PMID: 32109311 Free PMC article.
-
Sphingolipids in metabolic disease: The good, the bad, and the unknown.
Green CD, Maceyka M, Cowart LA, Spiegel S. Green CD, et al. Cell Metab. 2021 Jul 6;33(7):1293-1306. doi: 10.1016/j.cmet.2021.06.006. Cell Metab. 2021. PMID: 34233172 Free PMC article. Review.
-
"Dicing and Splicing" Sphingosine Kinase and Relevance to Cancer.
Haddadi N, Lin Y, Simpson AM, Nassif NT, McGowan EM. Haddadi N, et al. Int J Mol Sci. 2017 Sep 2;18(9):1891. doi: 10.3390/ijms18091891. Int J Mol Sci. 2017. PMID: 28869494 Free PMC article. Review.
-
The roles of bile acids and sphingosine-1-phosphate signaling in the hepatobiliary diseases.
Nagahashi M, Yuza K, Hirose Y, Nakajima M, Ramanathan R, Hait NC, Hylemon PB, Zhou H, Takabe K, Wakai T. Nagahashi M, et al. J Lipid Res. 2016 Sep;57(9):1636-43. doi: 10.1194/jlr.R069286. Epub 2016 Jul 26. J Lipid Res. 2016. PMID: 27459945 Free PMC article. Review.
References
-
- Lewis JR, Mohanty SR. Nonalcoholic fatty liver disease: a review and update. Dig Dis Sci. 2010;55:560–578. - PubMed
-
- Malaguarnera M, Di Rosa M, Nicoletti F, Malaguarnera L. Molecular mechanisms involved in NAFLD progression. J Mol Med (Berl) 2009;87:679–695. - PubMed
-
- Younossi ZM, Stepanova M, Afendy M, Fang Y, Younossi Y, Mir H, Srishord M. Changes in the prevalence of the most common causes of chronic liver diseases in the United States from 1988 to 2008. Clin Gastroenterol Hepatol. 2011;9:524–530. e521. quiz e560. - PubMed
-
- Almeda-Valdes P, Cuevas-Ramos D, Aguilar-Salinas CA. Metabolic syndrome and non-alcoholic fatty liver disease. Ann Hepatol. 2009;8 (Suppl 1):S18–24. - PubMed
-
- Polyzos SA, Kountouras J, Zavos C. Nonalcoholic fatty liver disease: the pathogenetic roles of insulin resistance and adipocytokines. Curr Mol Med. 2009;9:299–314. - PubMed
Publication types
MeSH terms
Substances
Grants and funding
LinkOut - more resources
Full Text Sources
Other Literature Sources
Miscellaneous