Primal eukaryogenesis: on the communal nature of precellular States, ancestral to modern life - PubMed
- ️Sun Jan 01 2012
Primal eukaryogenesis: on the communal nature of precellular States, ancestral to modern life
Richard Egel. Life (Basel). 2012.
Abstract
This problem-oriented, exploratory and hypothesis-driven discourse toward the unknown combines several basic tenets: (i) a photo-active metal sulfide scenario of primal biogenesis in the porespace of shallow sedimentary flats, in contrast to hot deep-sea hydrothermal vent conditions; (ii) an inherently complex communal system at the common root of present life forms; (iii) a high degree of internal compartmentalization at this communal root, progressively resembling coenocytic (syncytial) super-cells; (iv) a direct connection from such communal super-cells to proto-eukaryotic macro-cell organization; and (v) multiple rounds of micro-cellular escape with streamlined reductive evolution-leading to the major prokaryotic cell lines, as well as to megaviruses and other viral lineages. Hopefully, such nontraditional concepts and approaches will contribute to coherent and plausible views about the origins and early life on Earth. In particular, the coevolutionary emergence from a communal system at the common root can most naturally explain the vast discrepancy in subcellular organization between modern eukaryotes on the one hand and both archaea and bacteria on the other.
Figures
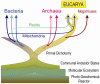
Recasting the early phylogenetic tree of life. The diagram emphasizes an unbroken chain of communal evolution from photo-geochemical reactors and organic molecular ecosystems to complex eukaryotic macro-cells. From the common matrix of communal ancestor states (here conceived as syncytial-like super-cells), prokaryotic micro-cells and acellular viral lineages "escaped" multiple times, as based on partly overlapping sampling of unified genomes from a highly redundant communal gene pool and subsequent clonal propagation. The primal dichotomy between bacterial and archaeal/protoeukaryotic stemlines occurred at the level of communal ancestors. All the composite modern eukaryotes descend from an ancestor that had already adopted some fairly advanced bacteria as permanently integrated mitochondrial endosymbionts. Similarly, cyanobacterial endosymbionts were acquired as plastids by the diverging lineage of green plants.
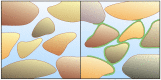
Porespace and mineral-facilitated biogenesis. In the multiply connected porespace between sedimented mineral grains (left panel), water can flow in various directions. Confluent layers of biogenic organic hydrogels (right panel, green) accumulate and progressively cover much of the mineral surface, whilst water can still move back and forth through most channels in the porespace network. The organic matrix does not only spread by incremental growth, but also by turbulent currents that redistribute the upper layers of the sediment. This diagram emphasizes the connectivity throughout a contiguous porespace. It does not, however, represent the nanoscale roughness and heterogeneity of the catalytically important mineral grains.
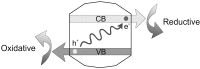
Photochemical charge separation, coupled to redox reactions. In a colloidal MeS particle (center), absorption of a UVB photon mobilizes an electron (e-) of the crystalline lattice into the energetic conductance band (CB). This leaves a complementary void in the lattice, which is conductable, too, as a hole (h+) in the valence band (VB). Both these 'bands' represent different levels on an energy scale. Upon reaching the particle surface, e- and h+ can initiate reductive and oxidative biochemical reaction pathways, respectively. Figure modified from Refs. [9,82].
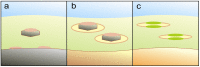
Tentative transition of energized electron transfer from mineral grains to membrane assemblages. (a) Peptide-rich micellar patches (pink), together with a rudimentary electron transfer chain, can have assembled at the substratum surface, between the precellular organic matrix (light green) and a larger external photoactive particle (grey), or in close contact with colloidal grains inside. (b) Fully enclosing such colloidal particles in membrane-bounded vesicles could render charge separation more effective. (c) Incorporating organic pigments (green) in the peptide-rich reaction centers could shift the effective bandwidth into the visible part of the sunlight spectrum.
Similar articles
-
The ancient Virus World and evolution of cells.
Koonin EV, Senkevich TG, Dolja VV. Koonin EV, et al. Biol Direct. 2006 Sep 19;1:29. doi: 10.1186/1745-6150-1-29. Biol Direct. 2006. PMID: 16984643 Free PMC article.
-
Koonin EV. Koonin EV. Biol Direct. 2006 Aug 14;1:22. doi: 10.1186/1745-6150-1-22. Biol Direct. 2006. PMID: 16907971 Free PMC article.
-
The changing view of eukaryogenesis - fossils, cells, lineages and how they all come together.
Dacks JB, Field MC, Buick R, Eme L, Gribaldo S, Roger AJ, Brochier-Armanet C, Devos DP. Dacks JB, et al. J Cell Sci. 2016 Oct 15;129(20):3695-3703. doi: 10.1242/jcs.178566. Epub 2016 Sep 26. J Cell Sci. 2016. PMID: 27672020 Review.
-
Bell PJ. Bell PJ. Ann N Y Acad Sci. 2009 Oct;1178:91-105. doi: 10.1111/j.1749-6632.2009.04994.x. Ann N Y Acad Sci. 2009. PMID: 19845630
-
Koonin EV. Koonin EV. Philos Trans R Soc Lond B Biol Sci. 2015 Sep 26;370(1678):20140333. doi: 10.1098/rstb.2014.0333. Philos Trans R Soc Lond B Biol Sci. 2015. PMID: 26323764 Free PMC article. Review.
Cited by
-
Cell Communications among Microorganisms, Plants, and Animals: Origin, Evolution, and Interplays.
Combarnous Y, Nguyen TMD. Combarnous Y, et al. Int J Mol Sci. 2020 Oct 28;21(21):8052. doi: 10.3390/ijms21218052. Int J Mol Sci. 2020. PMID: 33126770 Free PMC article. Review.
-
Origins and emergent evolution of life: the colloid microsphere hypothesis revisited.
Egel R. Egel R. Orig Life Evol Biosph. 2014 Apr;44(2):87-110. doi: 10.1007/s11084-014-9363-8. Epub 2014 Sep 11. Orig Life Evol Biosph. 2014. PMID: 25208738
-
Coenzyme world model of the origin of life.
Sharov AA. Sharov AA. Biosystems. 2016 Jun;144:8-17. doi: 10.1016/j.biosystems.2016.03.003. Epub 2016 Mar 9. Biosystems. 2016. PMID: 26968100 Free PMC article. Review.
-
If the cap fits, wear it: an overview of telomeric structures over evolution.
Fulcher N, Derboven E, Valuchova S, Riha K. Fulcher N, et al. Cell Mol Life Sci. 2014 Mar;71(5):847-65. doi: 10.1007/s00018-013-1469-z. Epub 2013 Sep 17. Cell Mol Life Sci. 2014. PMID: 24042202 Free PMC article. Review.
-
Life's Order, Complexity, Organization, and Its Thermodynamic-Holistic Imperatives.
Egel R. Egel R. Life (Basel). 2012 Nov 13;2(4):323-63. doi: 10.3390/life2040323. Life (Basel). 2012. PMID: 25371269 Free PMC article.
References
-
- Peretó J. Controversies on the origin of life. Int. Microbiol. 2005;8:23–31. - PubMed
-
- Pascal R., Boiteau L., Forterre P., Gargaud M., Lazcano A., Lopez-Garcia P., Maurel M.C., Moreira D., Peretó J., Prieur D., Reisse J. Prebiotic chemistry-biochemistry-emergence of life (4.4-2 Ga) Earth Moon Planets. 2006;98:153–203. doi: 10.1007/s11038-006-9089-3. - DOI
-
- Egel R. Integrative perspectives: In quest of a coherent framework for origins of life on earth. In: Egel R., Lankenau D.-H., Mulkidjanian A.Y., editors. Origins of Life: The Primal Self-Organization. Springer-Verlag; Heidelberg, Germany: 2011. pp. 289–360.
LinkOut - more resources
Full Text Sources