An integrative analysis reveals coordinated reprogramming of the epigenome and the transcriptome in human skeletal muscle after training - PubMed
An integrative analysis reveals coordinated reprogramming of the epigenome and the transcriptome in human skeletal muscle after training
Maléne E Lindholm et al. Epigenetics. 2014 Dec.
Abstract
Regular endurance exercise training induces beneficial functional and health effects in human skeletal muscle. The putative contribution to the training response of the epigenome as a mediator between genes and environment has not been clarified. Here we investigated the contribution of DNA methylation and associated transcriptomic changes in a well-controlled human intervention study. Training effects were mirrored by significant alterations in DNA methylation and gene expression in regions with a homogeneous muscle energetics and remodeling ontology. Moreover, a signature of DNA methylation and gene expression separated the samples based on training and gender. Differential DNA methylation was predominantly observed in enhancers, gene bodies and intergenic regions and less in CpG islands or promoters. We identified transcriptional regulator binding motifs of MRF, MEF2 and ETS proteins in the proximity of the changing sites. A transcriptional network analysis revealed modules harboring distinct ontologies and, interestingly, the overall direction of the changes of methylation within each module was inversely correlated to expression changes. In conclusion, we show that highly consistent and associated modifications in methylation and expression, concordant with observed health-enhancing phenotypic adaptations, are induced by a physiological stimulus.
Keywords: 450k arrays; CS, citrate synthase; CpG, cytosine-guanine dinucleotide; DEG, differentially expressed gene; DMP, differentially methylated position; DNA methylation; FDR, false discovery rate; LUMA, luminometric methylation assay; MDS, multidimensional scaling; MEF, myocyte enhancer factor; MRF, myogenic regulatory factor; PCA, principal component analysis; environment; epigenomics; exercise; integrative analysis; lifestyle; transcriptional network; β-HAD, 3-hydroxyacyl-CoA dehydrogenase.
Figures
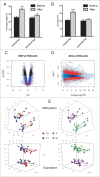
Effects of endurance exercise training are mirrored by alterations in DNA methylation and gene expression. The significant (* = P < 10−4) effect of training in the trained leg is shown by the increase in a 15 min performance test (A) and the citrate synthase activity in muscle biopsies (B) in T2 (after training) vs. T1 (before training). For comparison, the untrained leg is also shown, where a smaller change is observed in performance and no change in CS activity. # indicates significant differences between the changes in the 2 legs. Additional physiological measurements are shown in Figure S1B-C. The physiological changes are mirrored by modifications in DNA methylation (C) and gene expression (D). For DNA methylation, the effect size is measured as the difference in M-values and points in black correspond to DMPs with FDR < 0.05. For gene expression, the log2 (Fold Change) is plotted against the average log2(Counts Per Million) and red points correspond to genes with FDR <0.05. Correlation between changes in DNA methylation and CS activity exists and results are shown in Figure S5. The clustering of the samples is shown in (E) using either DNA methylation (upper panels) or gene expression (lower panels). A segment connects to measurements from the same subject obtained before and after training. Samples are alternatively colored by group (T1 = blue, T2 = red) or by gender (M = green, F = magenta). For DNA methylation, Principal Component Analysis was employed using only autosomal DMPs, while for gene expression the top 1000 genes with largest biological variation were chosen and the biological coefficient of variation used to produce a multidimensional scaling plot.
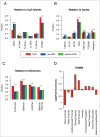
DNA methylation changes are primarily localized in enhancers. (A–C) For each annotation category, the relative fraction of positions located within each feature type is calculated for DMPs (red bars), non-DMPs (blue bars) and the entire position on the array (green bar). Panels A-B were obtained using Illumina annotation, while for panel C we used Illumina annotation (Enhancers) or publicly available ChIP-seq experiments on HSMM cells from which we derived H3K4me1 and H3K27ac peaks. The presence of H3K27ac mark denotes an active regulatory element, while H3K4me1+H3K27ac marks active enhancers and other distal elements. (D) The log2 fold enrichment for DMPs vs. the array was calculated for the relative fraction of probes falling in each category; data from chromatin segmentation algorithms in HSMM cells were used. For additional information, see Supplementary Methods S9. Significance codes: *P < 0.05; ***P < 0.001; Fisher's exact test.
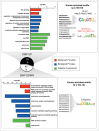
(See previous page). Muscle related processes and factors are enriched in the up-methylated DMPs. GREAT analysis was performed to retrieved functional categories associated with DMPs increasing or decreasing methylation after training. Up to top 5 categories passing the threshold (see Supplementary Methods S9) are shown for GO Molecular Function, Biological Process and Cellular Component. We tested the presence of known enriched motif on a symmetrical 200 bp window around each DMP (P < 10−10, consensus motif shown). Known profiles were clustered and a familial logo was drawn. For a corresponding ontology analysis of gene expression, see Figures S2-S3.
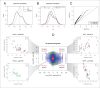
Identification of regions exhibiting positive or negative correlations between methylation and expression. (A) Distribution of Spearman correlation between DNA methylation and gene expression calculated either including all pairs of genes/methylation positions (gray line) or only pairs formed by a DMP and a DEG (black line). The 2 distributions are strongly different (P < 2.2e-16 Kolmogorov-Smirnov Test) and peaks of positive and negative correlation are highlighted after selecting changing sites after training. (B) The distribution of the Spearman correlation was stratified according to the genomic location of the DMP with respect to the linked gene. (C) A Q-Q plot was obtained by plotting the observed P-values from the above correlation analysis against those obtained under a uniform distribution. (D) A starburst plot illustrates the relationship between DNA methylation and expression changes, with a color density representation of the scatterplot of the pairs of genes/methylation positions. The horizontal axis is the FDR for each gene multiplied by the sign of the fold change, while the vertical axis is the FDR for methylation multiplied by the sign of the fold change. Green dots correspond to pairs where FDR<0.05. Numbers on each quadrant show the pairs (black) or genes (red) that fall in each region. Examples of expression/methylation pairs are given for the indicated probes and genes. Points represent samples and are colored as shown on the corresponding legends (training: T1 = black, T2 = red; gender: M = blue, F = red). The correlation for the cases taken from the significant quadrants is visually explained by the training, while the correlation obtained for non-significant cases may be explained because of the individual levels of methylation and gene expression being correlated at the subject level.
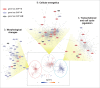
Transcriptional network analysis reveals coordinated alternations in methylation and gene expression. A transcriptional network was reconstructed using RNA expression data, showing 3 major domains (see Supplementary Methods S9 and Figure S7). The whole network is shown inside the rectangle at the bottom of the figure, where each node is colored according to the corresponding fold change. The 3 major domains (A, B, C) are zoomed and only genes with corresponding significant changes in DNA methylation are labeled. The color indicates whether the gene has corresponding DMPs that significantly increase (red) or decrease (blue) their methylation levels. A dark gray color indicates that the gene has significant but discordant changes in DNA methylation.
Similar articles
-
DNA methylation of exercise-responsive genes differs between trained and untrained men.
Geiger C, Needhamsen M, Emanuelsson EB, Norrbom J, Steindorf K, Sundberg CJ, Reitzner SM, Lindholm ME. Geiger C, et al. BMC Biol. 2024 Jul 4;22(1):147. doi: 10.1186/s12915-024-01938-6. BMC Biol. 2024. PMID: 38965555 Free PMC article.
-
Kanzleiter T, Jähnert M, Schulze G, Selbig J, Hallahan N, Schwenk RW, Schürmann A. Kanzleiter T, et al. Am J Physiol Endocrinol Metab. 2015 May 15;308(10):E912-20. doi: 10.1152/ajpendo.00289.2014. Epub 2015 Mar 24. Am J Physiol Endocrinol Metab. 2015. PMID: 25805191
-
Gorski PP, Raastad T, Ullrich M, Turner DC, Hallén J, Savari SI, Nilsen TS, Sharples AP. Gorski PP, et al. FASEB J. 2023 Jan;37(1):e22720. doi: 10.1096/fj.202201510RR. FASEB J. 2023. PMID: 36542473
-
Brown WM. Brown WM. Br J Sports Med. 2015 Dec;49(24):1567-78. doi: 10.1136/bjsports-2014-094073. Epub 2015 Mar 30. Br J Sports Med. 2015. PMID: 25824446 Review.
-
Physical Exercise and Epigenetic Modifications in Skeletal Muscle.
Widmann M, Nieß AM, Munz B. Widmann M, et al. Sports Med. 2019 Apr;49(4):509-523. doi: 10.1007/s40279-019-01070-4. Sports Med. 2019. PMID: 30778851 Review.
Cited by
-
Brun JF, Myzia J, Varlet-Marie E, Raynaud de Mauverger E, Mercier J. Brun JF, et al. Nutrients. 2022 Apr 12;14(8):1605. doi: 10.3390/nu14081605. Nutrients. 2022. PMID: 35458167 Free PMC article. Review.
-
Genome-wide epigenetic modifications in sports horses during training as an adaptation phenomenon.
Cappelli K, Mecocci S, Porceddu A, Albertini E, Giontella A, Miglio A, Silvestrelli M, Verini Supplizi A, Marconi G, Capomaccio S. Cappelli K, et al. Sci Rep. 2023 Nov 1;13(1):18786. doi: 10.1038/s41598-023-46043-w. Sci Rep. 2023. PMID: 37914824 Free PMC article.
-
Sex differences in muscle protein expression and DNA methylation in response to exercise training.
Landen S, Jacques M, Hiam D, Alvarez-Romero J, Schittenhelm RB, Shah AD, Huang C, Steele JR, Harvey NR, Haupt LM, Griffiths LR, Ashton KJ, Lamon S, Voisin S, Eynon N. Landen S, et al. Biol Sex Differ. 2023 Sep 5;14(1):56. doi: 10.1186/s13293-023-00539-2. Biol Sex Differ. 2023. PMID: 37670389 Free PMC article.
-
Temporal dynamics of the multi-omic response to endurance exercise training.
MoTrPAC Study Group; Lead Analysts; MoTrPAC Study Group. MoTrPAC Study Group, et al. Nature. 2024 May;629(8010):174-183. doi: 10.1038/s41586-023-06877-w. Epub 2024 May 1. Nature. 2024. PMID: 38693412 Free PMC article.
References
-
- Matheson GO, Klügl M, Engebretsen L, Bendiksen F, Blair SN, Börjesson M, Budgett R, Derman W, Erdener U, Ioannidis JPA, et al. . Prevention and management of non-communicable disease: the IOC consensus statement, Lausanne 2013. Sports Med 2013; 43:1075-88; PMID:24129783; http://dx.doi.org/10.1007/s40279-013-0104-3 - DOI - PubMed
-
- Coffey VG, Hawley JA. The Molecular Bases of Training Adaptation. Sports Med 2007; 37:737-63; PMID:17722947; http://dx.doi.org/10.2165/00007256-200737090-00001 - DOI - PubMed
-
- Jones PA. Functions of DNA methylation: islands, start sites, gene bodies and beyond. Nat Rev Genet 2012; 13:484-92; PMID:22641018; http://dx.doi.org/10.1038/nrg3230 - DOI - PubMed
-
- Heyn H, Esteller M. DNA methylation profiling in the clinic: applications and challenges. Nat Rev Genet 2012; 13:679-92; PMID:22945394; http://dx.doi.org/10.1038/nrg3270 - DOI - PubMed
-
- Jones PA, Baylin SB. The epigenomics of cancer. Cell 2007; 128:683-92; PMID:17320506; http://dx.doi.org/10.1016/j.cell.2007.01.029 - DOI - PMC - PubMed
Publication types
MeSH terms
LinkOut - more resources
Full Text Sources
Other Literature Sources
Medical
Molecular Biology Databases
Research Materials
Miscellaneous