Role of the clathrin adaptor PICALM in normal hematopoiesis and polycythemia vera pathophysiology - PubMed
. 2015 Apr;100(4):439-51.
doi: 10.3324/haematol.2014.119537. Epub 2014 Dec 31.
Manami Maeda 2 , Mithun Pasham 3 , Francois Aguet 4 , Silvia K Tacheva-Grigorova 3 , Takeshi Masuda 5 , Hai Yi 6 , Sung-Uk Lee 2 , Jian Xu 7 , Julie Teruya-Feldstein 8 , Maria Ericsson 4 , Ann Mullally 5 , John Heuser 9 , Tom Kirchhausen 3 , Takahiro Maeda 10
Affiliations
- PMID: 25552701
- PMCID: PMC4380716
- DOI: 10.3324/haematol.2014.119537
Role of the clathrin adaptor PICALM in normal hematopoiesis and polycythemia vera pathophysiology
Yuichi Ishikawa et al. Haematologica. 2015 Apr.
Abstract
Clathrin-dependent endocytosis is an essential cellular process shared by all cell types. Despite this, precisely how endocytosis is regulated in a cell-type-specific manner and how this key pathway functions physiologically or pathophysiologically remain largely unknown. PICALM, which encodes the clathrin adaptor protein PICALM, was originally identified as a component of the CALM/AF10 leukemia oncogene. Here we show, by employing a series of conditional Picalm knockout mice, that PICALM critically regulates transferrin uptake in erythroid cells by functioning as a cell-type-specific regulator of transferrin receptor endocytosis. While transferrin receptor is essential for the development of all hematopoietic lineages, Picalm was dispensable for myeloid and B-lymphoid development. Furthermore, global Picalm inactivation in adult mice did not cause gross defects in mouse fitness, except for anemia and a coat color change. Freeze-etch electron microscopy of primary erythroblasts and live-cell imaging of murine embryonic fibroblasts revealed that Picalm function is required for efficient clathrin coat maturation. We showed that the PICALM PIP2 binding domain is necessary for transferrin receptor endocytosis in erythroblasts and absolutely essential for erythroid development from mouse hematopoietic stem/progenitor cells in an erythroid culture system. We further showed that Picalm deletion entirely abrogated the disease phenotype in a Jak2(V617F) knock-in murine model of polycythemia vera. Our findings provide new insights into the regulation of cell-type-specific transferrin receptor endocytosis in vivo. They also suggest a new strategy to block cellular uptake of transferrin-bound iron, with therapeutic potential for disorders characterized by inappropriate red blood cell production, such as polycythemia vera.
Copyright© Ferrata Storti Foundation.
Figures
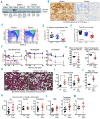
Picalm knockout mice develop microcytic and hypochromic anemia. (A) Picalm is predominantly expressed in erythroid cells. Mouse hematopoietic cells from bone marrow (BM), spleen or thymus were FACS-sorted using lineage-specific surface markers. Picalm protein levels were analyzed by western blot using anti-Picalm antibody. (B) Immunohistochemical analysis for Picalm was performed on formalin-fixed, paraffin-embedded fetal liver (FL) sections of 13.5 d.p.c. embryos. Brown stains in WT FL represent Picalm protein. Complete absence of Picalm protein in Picalm−/−FL confirmed successful Picalm deletion in vivo. Insets: high magnification images. (C) Erythroid development in FL was analyzed by FACS based on surface expression levels of TER119 and CD71 (TfR). Each gate (R1 to R5) was determined as described. Representative FACS profiles of Picalm+/+ or Picalm−/− FL cells from 14.5 d.p.c. embryos are shown. (D) Dot graph shows proportions of R4 erythroblasts (TER119+CD71dim+), which consist mainly of ortho-chromatophilic erythroblasts and reticulocytes. Horizontal black bars: average value; error bars: standard deviation. (E) Absolute counts of 14.5 d.p.c. FL cells, which consist mainly of erythroid lineage cells. Horizontal black bars: average value; error bars: standard deviation. (F) Time-course analysis of peripheral blood (PB) upon pIpC injection. Three pIpC doses (250 mg/injection) were administered intraperitoneally as described elsewhere. PB counts were examined at indicated time points after injections. PicalmF/F Mx1Cre+ mice, but not PicalmF/+ Mx1Cre+ or control mice (PicalmF/+ or PicalmF/F), exhibited a marked reduction in red blood cell counts, hemoglobin and hematocrit. Each plot represents average values of 10–18 mice; error bars: standard deviation. (G) Dot graphs represent average red blood cell size and average amount of hemoglobin per red blood cell 3 months after pIpC injections. Error bars: standard deviation. (H) PB smears stained with benzidine, which stains hemoglobin. Benzidine-negative/dim red blood cells, which contain low concentrations of intracellular hemoglobin, are evident in PB smears of PicalmF/F Mx1Cre+ mice. Sizes and shape of Picalm-deficient red blood cells are more variable than those of control samples. (I) Proportions (left) and absolute counts (right) of reticulocytes 3 months after pIpC injections. Horizontal black bars: average value; error bars: standard deviation. (J) Red cell distribution width (RDW), a measure of deviation in red blood cell volume in PB, was markedly increased in PicalmF/F Mx1Cre+ mice. RDW was measured 3 months after pIpC injections. Horizontal black bars: average value; error bars: standard deviation. (K) Levels of iron (Fe), ferritin and transferrin in serum were measured 2 months after pIpC injections. Serum Fe levels in PicalmF/F Mx1Cre+ mice were higher than those of controls, while ferritin and transferrin levels were comparable to those in controls. Horizontal black bars: average value; error bars: standard deviation. (L) Total iron binding capacity (TIBC) and transferrin saturation (%). Horizontal black bars: average value; error bars: standard deviation. (M) Platelets counts 3 months after pIpC injections. Horizontal black bars: average value; error bars: standard deviation.
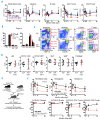
Picalm is dispensable for myeloid and B-lymphoid development. (A) Time-course analysis of non-erythroid cells in peripheral blood upon pIpC injection. White blood cell counts were examined at indicated time points after injections. Proportions of myeloid and lymphoid cells were determined by FACS. Each plot represents average value of 10–18 mice; error bars: standard deviation. (B) Graph shows frequencies of CD4/8 double-negative (DN), CD4/8 double-positive (DP), CD4 single-positive (CD4SP) and CD8 single-positive (CD8SP) T cells 2 months after pIpC injections (left). Frequencies of DN fractions (DN1: CD44+CD25−, DN2: CD44+CD25+, DN3: CD44−CD25+ and DN4: CD44−CD25−) are also shown (right). (C) Representative FACS profiles of bone marrow cells from control (PicalmF/F) and Picalm knockout mice (PicalmF/F Mx1Cre+) 2 months after pIpC injections. (D) Dot graphs of absolute counts (per leg) of hematopoietic stem/progenitors in bone marrow 2 months after pIpC injections. Stem/progenitors were identified by FACS as described elsewhere. LSK (lineage-Sca1+c-Kit+); HSC (hematopoietic stem cells); MPP (multi-potential progenitors); MkP (megakaryocyte progenitors); GMP: granulocyte macrophage progenitors; PreMegE (pre-megakaryocyte–erythrocyte progenitors). Horizontal black bars: average value; error bars: standard deviation. (E) Schematic representation of a bone marrow transplant experiment. One million bone marrow mononuclear cells (BMMNC) from either control (PicalmF/F) or knockout (PicalmF/F Mx1Cre+) mice were transferred to a lethally-irradiated (9 Gy, single dose) recipient mouse along with CD45.1+ 2 × 105 BMMNC (competitors). Recipients were injected with pIpC 1 month after transplantation (250 mg × 3). (F) Peripheral blood counts were analyzed by a hematology analyzer and FACS 1, 3 and 12 months after pIpC injections. Each dot represents the average value of 12 samples in each group; error bars: standard deviation.
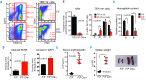
Inefficient erythroid development in PicalmF/F Mx1Cre+ mice. (A) Representative FACS profiles of splenic erythroblasts from control (PicalmF/F) and Picalm knockout mice (PicalmF/F Mx1Cre+) 2 months after pIpC injections. Immature erythroblasts (R2) did not efficiently give rise to mature erythrocytes (R4). (B) Bar graphs show proportions of R4 erythroblasts in the spleen 1 month after pIpC injections (left). Proportions of CD44/FSC-based sub-fractions are also shown (right). Histograms: average value of three samples per genotype; error bars: standard deviation. (C) Benzidine staining of splenic erythroblasts. FACS-sorted erythroblasts were cyto-spun onto glass slides and stained with benzidine, an indicator of intracellular hemoglobin levels. Graph shows frequencies of benzidine-positive cells in stage II and III cells. Almost all cells at stage III were benzidine-positive in control cells, while only 20% of Picalm-deficient erythroblasts stained positive. Histograms: average value of three samples per genotype; error bars: standard deviation. (D) Apoptosis of R2 erythroblasts was measured by FACS by appearance of cleaved PARP (left) and annexin V-staining (right) 2 months after pIpC injections. Histograms: average value of four samples per genotype; error bars: standard deviation. (E) Dot graph shows serum erythropoietin levels 2 months after pIpC injections. In response to hypoxia in the periphery, erythropoietin levels in Picalm knockout mice were 20-fold higher than those seen in controls. Horizontal black bars: average value; error bars: standard deviation. (F) Dot graphs represent average spleen weight 2 months after pIpC injections (left). Horizontal black bars: average value; error bars: standard deviation. Representative picture of spleens (right).
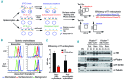
TfR endocytosis is impaired in Picalm-deficient erythroblasts. (A) FACS-based transferrin/TfR endocytosis assay. To measure the amount of internalized transferrin, splenocytes were incubated at 37°C for 8 min with Alexa-647 transferrin, chilled to 4°C (to stop endocytosis) and washed with low-pH buffer to remove transferrin bound to the cell surface. For surface-bound transferrin, cells were incubated at 4°C with Alexa-647 transferrin and then washed with neutral pH buffer. Samples were then incubated with fluorescently-tagged antibodies specific for erythroid and lymphoid cells and subjected to FACS analysis. As a background control, splenocytes were incubated with at 4°C with Alexa-647 transferrin for 8 min, washed with acid buffer and analyzed by FACS. Efficiency of transferrin (Tf) endocytosis was calculated by dividing mean fluorescence intensity (MFI) of internalized transferrin by that of surface-bound transferrin. (B) Representative FACS profiles of transferrin/TfR endocytosis assay in erythroblasts. MFI of internalized- (blue lines) and surface bound- (red lines) transferrin was measured by FACS at different stages of erythroid differentiation. Background signals are also presented for each population (green lines). Red-dashed lines indicate levels of surface-bound Alexa-transferrin in control erythroblasts. (C) Bar graphs show efficiencies of transferrin endocytosis in basophilic and polychromatophilic erythroblasts. Experiments were repeated three times, and representative results are presented. Histograms: average value of two samples per genotype; error bars: standard deviation. (D) Western blot for TfR in FACS-sorted erythroblasts. Samples from two mice per genotype (control: #1 and #2, Picalm knockout: #3 and #4) were analyzed. TfR protein levels in Picalm-deficient erythroblasts were comparable to those of controls. Western blot for H-ferritin, indicative of intracellular iron storage; Picalm and Tubulin are also shown. Mφ: protein lysates of bone marrow macrophages. Asterisk: non-specific signal.
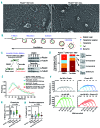
Formation of clathrin-coated vesicles in Picalm-deficient cells. (A) Representative pictures of freeze-etch electron microscopy in primary splenic erythroblasts. Formation of mature vesicles was barely observed in Picalm-deficient erythroblasts. Arrows indicate clathrin-coated vesicles. (B) Formation of endocytic clathrin-coated structures on the plasma membrane. The process of clathrin-dependent endocytosis is dynamic and has multiple steps, including initiation, maturation, scission and uncoating. (C) Generation of mouse embryonic fibroblasts (MEF) in which Picalm is inducibly deleted by tamoxifen treatment (PicalmF/F ERT2-Cre+ MEF). MEF were established from a 13.5 d.p.c PicalmF/F ERT2-Cre+ embryo, immortalized with large T antigen and retrovirally transduced with EGFP-tagged AP2-bound adaptin σ2 (σ2-EGFP)., Western blot for Picalm confirmed inducible depletion of Picalm protein in tamoxifen-treated but not control DMSO-treated MEF (right). Two splice forms of Picalm (Picalm-long and -short) were evident in MEF, and both were absent after tamoxifen treatment. (D) Relative frequency of each clathrin structure was measured 72 h after tamoxifen (or DMSO) treatment of PicalmF/F ERT2-Cre MEF. The proportion of mature vesicles was significantly reduced in tamoxifen-treated (Picalm-deleted) MEF. (E) Summary of the various cell types used to acquire live-cell imaging data using spinning-disk confocal microscopy of PicalmF/F ERT2-Cre+ MEF cells stably expressing AP2 (σ2-EGFP). Picalm KO denotes Picalm deletion by tamoxifen addition; Ap180 KD denotes Ap180 knockdown by shRNA. The general statistics indicate the number of cells analyzed per condition as well as the average ± SD of coated pits (events) per cell and the total number of events detected using the tracking algorithm described by Aguet et al. Coated pits are structures that gained fluorescence intensity relative to the first detected time point. The plot shows the lifetime distributions for coated pits. (F) Plots corresponding to the average fluorescence intensities from the corresponding number of cells, calculated from productive coated pit trajectories binned by lifetime represented as mean intensity (solid lines) and standard error (shaded areas). The top of each graph indicates the average percentage of coated pits within each cohort. (G) The panel corresponds to the area density of coated pits that formed per min during the 5 min duration of the time-series. (H) The panel shows the density of persistent structures, defined as structures present for the full duration of the time-series. Box plots show median, 25th, and 75th percentiles, and outermost data points. Statistical significance was determined using the permutation test for medians.
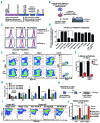
PICALM/PIP2 interaction is critical for erythroid differentiation. (A) Structure of human PICALM protein and predicted domains/motifs. Exon 13 is spliced out in the PICALM short form. Every mutant defined at the top was tested for its capacity to rescue PICALM deficiency. Mutated residues in each mutant are described in Online Supplementary Figure S4D. (B) Experimental outline of “add-back” rescue experiments using PICALM-knockdown K562 leukemia cells (left). Lentivirus vectors encoding shRNA targeting the PICALM 3′UTR were generated, and knockdown efficiency in K562 leukemia cells was tested by western blot. K562 cells stably expressing PICALM shRNA#1 were used for the experiment (right). Each PICALM mutant was retrovirally “added-back” into PICALM-knockdown K562 cells and transferrin uptake analyzed 4 days after transduction. Retrovirus also encodes a mCherry fluorescent protein, allowing tracking of cells expressing mutant or WT PICALM. (C) FACS-based transferrin/TfR endocytosis assays were performed as described. Representative FACS profiles of empty vector-, WT-PICALM (long form)- and PIP2-PICALM- infected cells are shown. (D) Efficiency of transferrin endocytosis in mCherry-positive cells was calculated as described in Figure 4A. Histograms show the relative efficiency of transferrin endocytosis upon “add-back” of empty vector or PICALM mutants. Transferrin endocytosis was significantly reduced in empty- or PIP2-PICALM-transduced cells compared to that of WT-PICALM-transduced cells. In contrast, other PICALM mutants enhanced TfR endocytosis in PICALM-knockdown K562 cells up to levels seen with WT-PICALM. Histograms: average value of two to four samples per condition; error bars: standard deviation. (E) Erythroid development in culture was examined daily for 3 days after induction of erythroid differentiation. Representative FACS profiles of control-and Picalm-deficient cells are shown. The extent of erythroid differentiation was assessed by FACS based on surface marker expression levels (TER119 and CD71) and cell size [forward scatter (FSC) and side scatter (SSC)]. R4 cells (TER119+CD71dim+), which consist mainly of ortho-chromatophilic erythroblasts and reticulocytes, are depicted with red rectangles. Fully-differentiated erythroid cells are smaller than immature erythroblasts and fall into the FSClow SSClow fraction. (F) Bar graphs show frequencies of mature erythroblasts after 3 days of culture. Picalm-deficient stem/progenitors gave rise to markedly fewer mature erythroblasts compared to controls. Experiments were repeated at least four times, and representative results are presented. Histograms: average value of two samples per genotype; error bars: standard deviation. (G) Iron supplement treatment (ferric ammonium citrate: FAC) significantly enhanced erythroid development of Picalm-deficient progenitors in a dose-dependent manner. Bar graphs show cell counts of each fraction (R0–R4) on day 2 in the presence or absence of FAC in the culture medium. Experiments were repeated three times, and representative results are presented. (H) Functional rescue experiments. Bone marrow stem/progenitor cells were obtained from PicalmF/F Mx1Cre+ mice 2 months after pIpC injections and transduced with retrovirus vectors encoding WT- or PIP2-PICALM. Transduced cells were subsequently induced for erythroid differentiation as described. The extent of erythroid differentiation was examined by FACS within GFP-positive (transduced) fractions. (I) Representative FACS profiles of WT- or PIP2-PICALM-transduced cells after 3 days of culture. FACS profiles for GFP-positive fractions are shown. WT-PICALM fully rescued Picalm deficiency in erythroid development: greater than 40% of GFP-positive (WT-PICALM-transduced) cells were mature erythroblasts on day 3, while PIP2-PICALM (or PH-PICALM)-transduced cells were not rescued. (J) Histograms show proportions of mature erythroblasts (%R4 and %FSClow SSClow) in empty vector-, PIP2- or WT-PICALM-transduced cells after 3 days of culture. Experiments were repeated three times, and representative results are presented. Histograms: average value of two samples per condition; error bars: standard deviation.
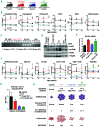
Picalm deletion ameliorates PV pathophysiology in mice. (A) Hematopoietic-specific Picalm conditional knockout mice were bred with PV mice (Jak2+/V617FVav-Cre+) to generate Picalm-deficient PV mice (PicalmF/FJak2+/V617FVav-Cre+). Four indicated groups were analyzed. (B) Time-course analysis of peripheral blood. Picalm-deficient PV mice (green line) exhibited a marked reduction in red blood cell counts, hemoglobin and hematocrit compared to PV mice (red line). Graphs represent average values of three of eight mice for each genotype. Error bars: standard deviation. (C) Total RNA was extracted from PB without red blood cell lysis and cDNA was prepared using standard methodology. Expression of WT and mutant Jak2 (Jak2 V617F) mRNA was analyzed by reverse transcription polymerase chain reaction (RT-PCR) using allele-specific primers as described. Mutant Jak2 transcripts were detected only in PB samples from PV and Picalm-deficient PV mice. RT-PCR for the housekeeping genes β-actin and Hprt was also performed. A representative gel is shown. (D) Jak2 kinase activity was examined by western blotting for the phosphorylated form of Stat5 (p-Stat5). Splenic erythroblasts were serum-starved for 2 h, treated with erythropoietin (EPO) for 10 min and protein extracts prepared. Western blot for Jak2 and Stat5 are also shown. Hsp90: loading control. Splenic erythroblasts of control mice were obtained after phenylhydrazine (PHZ) treatment. (E) mRNA levels of Bcl-XL in splenic erythroblasts were examined by realtime quantitative RT-PCR. (F) Time-course analysis of myeloid and lymphoid cell counts in PB. (G) Endocytic rate of transferrin/TfR in splenic erythroblasts was measured by FACS as described. (H) The Jak2 V617F mutation causes constitutive activation of the Jak/Stat pathway in erythroid progenitors, leading to expansion of immature erythroblasts. PV mice exhibit a relative iron deficiency due to erythroid cell overproduction. In Picalm knockout (KO) mice, serum erythropoietin (EPO) levels are high and EPO/EPOR-mediated Jak/Stat signals are activated in erythroblasts; however, erythropoiesis (mature red cell production) is inefficient as TfR-mediated cellular iron uptake is impaired. In Picalm-deficient PV mice, regardless of Jak2 V617F-driven Jak2/Stat5 activation, Picalm deficiency limits iron availability in erythroblasts, blocking red cell overproduction.
Similar articles
-
Suzuki M, Tanaka H, Tanimura A, Tanabe K, Oe N, Rai S, Kon S, Fukumoto M, Takei K, Abe T, Matsumura I, Kanakura Y, Watanabe T. Suzuki M, et al. PLoS One. 2012;7(2):e31854. doi: 10.1371/journal.pone.0031854. Epub 2012 Feb 21. PLoS One. 2012. PMID: 22363754 Free PMC article.
-
The PICALM protein plays a key role in iron homeostasis and cell proliferation.
Scotland PB, Heath JL, Conway AE, Porter NB, Armstrong MB, Walker JA, Klebig ML, Lavau CP, Wechsler DS. Scotland PB, et al. PLoS One. 2012;7(8):e44252. doi: 10.1371/journal.pone.0044252. Epub 2012 Aug 30. PLoS One. 2012. PMID: 22952941 Free PMC article.
-
Klebig ML, Wall MD, Potter MD, Rowe EL, Carpenter DA, Rinchik EM. Klebig ML, et al. Proc Natl Acad Sci U S A. 2003 Jul 8;100(14):8360-5. doi: 10.1073/pnas.1432634100. Epub 2003 Jun 27. Proc Natl Acad Sci U S A. 2003. PMID: 12832620 Free PMC article.
-
Polycythemia vera: scientific advances and current practice.
Tefferi A, Spivak JL. Tefferi A, et al. Semin Hematol. 2005 Oct;42(4):206-20. doi: 10.1053/j.seminhematol.2005.08.003. Semin Hematol. 2005. PMID: 16210034 Review.
-
PICALM and Alzheimer's Disease: An Update and Perspectives.
Ando K, Nagaraj S, Küçükali F, de Fisenne MA, Kosa AC, Doeraene E, Lopez Gutierrez L, Brion JP, Leroy K. Ando K, et al. Cells. 2022 Dec 10;11(24):3994. doi: 10.3390/cells11243994. Cells. 2022. PMID: 36552756 Free PMC article. Review.
Cited by
-
Epigenetic inactivation of ERF reactivates γ-globin expression in β-thalassemia.
Bao X, Zhang X, Wang L, Wang Z, Huang J, Zhang Q, Ye Y, Liu Y, Chen D, Zuo Y, Liu Q, Xu P, Huang B, Fang J, Lao J, Feng X, Li Y, Kurita R, Nakamura Y, Yu W, Ju C, Huang C, Mohandas N, Li D, Zhao C, Xu X. Bao X, et al. Am J Hum Genet. 2021 Apr 1;108(4):709-721. doi: 10.1016/j.ajhg.2021.03.005. Epub 2021 Mar 17. Am J Hum Genet. 2021. PMID: 33735615 Free PMC article.
-
CircCLTH promotes skeletal muscle development and regeneration.
Chen M, Liu Q, Song M, Liu X, Huang K, Zhong D, Chen Y, Jiang M, Sun J, Ouyang Y, Sooranna SR, Shi D, Li H. Chen M, et al. Epigenetics. 2022 Dec;17(13):2296-2317. doi: 10.1080/15592294.2022.2117115. Epub 2022 Sep 8. Epigenetics. 2022. PMID: 36043316 Free PMC article.
-
CALM supports clathrin-coated vesicle completion upon membrane tension increase.
Willy NM, Colombo F, Huber S, Smith AC, Norton EG, Kural C, Cocucci E. Willy NM, et al. Proc Natl Acad Sci U S A. 2021 Jun 22;118(25):e2010438118. doi: 10.1073/pnas.2010438118. Proc Natl Acad Sci U S A. 2021. PMID: 34155137 Free PMC article.
-
Creation of a functional unc-11/PICALM GFP knock-in by CRISPR.
Gallegos M, Hermes A, Patra D. Gallegos M, et al. MicroPubl Biol. 2021 Apr 28;2021:10.17912/micropub.biology.000389. doi: 10.17912/micropub.biology.000389. MicroPubl Biol. 2021. PMID: 33937722 Free PMC article.
-
Erythropoietin signaling regulates heme biosynthesis.
Chung J, Wittig JG, Ghamari A, Maeda M, Dailey TA, Bergonia H, Kafina MD, Coughlin EE, Minogue CE, Hebert AS, Li L, Kaplan J, Lodish HF, Bauer DE, Orkin SH, Cantor AB, Maeda T, Phillips JD, Coon JJ, Pagliarini DJ, Dailey HA, Paw BH. Chung J, et al. Elife. 2017 May 29;6:e24767. doi: 10.7554/eLife.24767. Elife. 2017. PMID: 28553927 Free PMC article.
References
-
- Ciechanover A, Schwartz AL, Dautry-Varsat A, Lodish HF. Kinetics of internalization and recycling of transferrin and the transferrin receptor in a human hepatoma cell line. Effect of lysosomotropic agents. J Biol Chem. 1983;258(16):9681–9689. - PubMed
Publication types
MeSH terms
Substances
LinkOut - more resources
Full Text Sources
Other Literature Sources
Molecular Biology Databases
Research Materials
Miscellaneous