Developing 3D SEM in a broad biological context - PubMed
doi: 10.1111/jmi.12211. Epub 2015 Jan 26.
A Kremer 1 2 3 , S Lippens 1 2 3 , S Bartunkova 1 2 3 , C Blanpain 5 , M Fendrych 6 7 8 , A Goossens 6 7 , M Holt 9 10 , S Janssens 2 11 12 , M Krols 2 4 , J-C Larsimont 5 , C Mc Guire 2 3 , M K Nowack 6 7 , X Saelens 2 3 , A Schertel 13 , B Schepens 2 3 , M Slezak 9 , V Timmerman 4 , C Theunis 14 15 , R VAN Brempt 14 15 , Y Visser 14 15 , C J Guérin 1 2 3
Affiliations
- PMID: 25623622
- PMCID: PMC4670703
- DOI: 10.1111/jmi.12211
Developing 3D SEM in a broad biological context
A Kremer et al. J Microsc. 2015 Aug.
Abstract
When electron microscopy (EM) was introduced in the 1930s it gave scientists their first look into the nanoworld of cells. Over the last 80 years EM has vastly increased our understanding of the complex cellular structures that underlie the diverse functions that cells need to maintain life. One drawback that has been difficult to overcome was the inherent lack of volume information, mainly due to the limit on the thickness of sections that could be viewed in a transmission electron microscope (TEM). For many years scientists struggled to achieve three-dimensional (3D) EM using serial section reconstructions, TEM tomography, and scanning EM (SEM) techniques such as freeze-fracture. Although each technique yielded some special information, they required a significant amount of time and specialist expertise to obtain even a very small 3D EM dataset. Almost 20 years ago scientists began to exploit SEMs to image blocks of embedded tissues and perform serial sectioning of these tissues inside the SEM chamber. Using first focused ion beams (FIB) and subsequently robotic ultramicrotomes (serial block-face, SBF-SEM) microscopists were able to collect large volumes of 3D EM information at resolutions that could address many important biological questions, and do so in an efficient manner. We present here some examples of 3D EM taken from the many diverse specimens that have been imaged in our core facility. We propose that the next major step forward will be to efficiently correlate functional information obtained using light microscopy (LM) with 3D EM datasets to more completely investigate the important links between cell structures and their functions.
Keywords: Correlative light and electron microscopy; focused ion beam scanning electron microscopy; sample preparation; serial block-face scanning electron microscopy.
© 2015 The Authors Journal of Microscopy © 2015 Royal Microscopical Society.
Figures
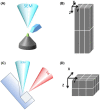
Schemes of serial block-face SEM techniques. (A) A schematic view of SBF-SEM: the electron beam scans the surface of the sample and sections (≥20 nm) are removed by a fully automated ultra-microtome. (B) Images acquired by SBF-SEM results in voxels with a larger z than x, y dimensions. (C) A schematic view of FIB-SEM: a Focused Ion Beam aligned to a coincidence point with the electron column (SEM) is used to create a surface for imaging with the electron beam, and subsequently to mill away sections ≥5 nm. (D) Thin sections in the FIB-SEM allow imaging at isotropic voxels, where x = y = z.
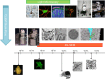
Summary of imaging modalities and scales. Diagram illustrating the imaging modalities and scales used in correlative microscopy. Making use of LM techniques that can yield large fields of view as well as high resolution allows for functional studies to be performed. Methods for preserving, preparing and transferring these samples to 3D SEM can be used to create a correlative microscopy work flow to better link cellular events to the underlying cellular nano-structure.
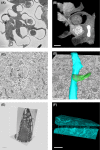
3D-EM images, acquired by SBF-SEM. (A) Mouse lung tissue imaged by SBF-SEM, X, Y pixels 37 nm, Z slices 70 nm. Single plane from 3D series showing lung alveoli and immune cells. Dark areas in left corners are caused by charging, a common issue when imaging bare resin. Scale bar: 5 μm. (B) Volume representation of the image stack made in Fiji. This view shows an immune cell residing in an alveolus. Total volume = 19,47 × 22,42 × 7,14 μm. Scale bars 5 μm. (C) Mouse brain imaged at 4000 × 4000 pixels, X, Y pixels 6.3 × 6.3 nm Z slices 40 nm. Scale bar: 5μm. (D) Manual segmentation of a subset (7,91 × 7,51 × 0,84 μm) of this dataset done using IMOD. Dendrites are presented in blue, a synapse in green and its synaptic vesicles in pink. Scale bar: 1μm. (E) Orthogonal views of 2000 slices at 75 nm Z of a 5-days-old seedling from Arabidopsis thaliana. Scale bar: 20 μm. (F) Arabidopsis root tip image stack of 10,35 × 7,23 × 3,9 μm. 3D volume reconstruction shows plasmodesmata that connect the cytoplasm of 2 neighboring cells. Scale bar: 20 μm.
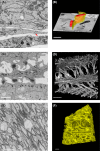
Examples of 3D-EM using FIB-SEM. (A) Mouse embryonic fibroblasts. A single image showing numerous subcellular organelles. The red arrowhead indicates a clathrin coated pit. Scale bar: 1 μm. (B) Manual segmentation of a dataset using IMOD consisting of 7,55 × 7,55 × 10 nm voxels. Reconstruction used to show the detailed geometry of the ER (yellow) and mitochondria (red). Scale bar: 1 μm. (C) Cultured lung epithelial cells from a dataset consisting of 6,2 × 6,2 × 15 nm voxels. A single image showing staining of intermediate filaments and desmosomes. Scale bar: 1 μm. (D) 3D volume rendering was done in Fiji showing the organization of the intermediate filaments and connections to the desmosomes. Scale bar: 1 μm. (E) Mouse brain tissue (corpus callosum) imaged with isotropic voxels (25 × 25 × 25 nm) from a dataset of 25 × 19 × 19 μm. Scale bar: 5 μm. (F) Volume reconstruction of a subset of the data (17 × 17 × 6,9 μm) was done in Fiji showing myelin sheaths running through the tissue. Scale bar: 5 μm.
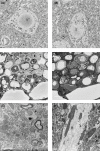
Developing new protocols for SBF-SEM. (A) Mouse brain tissue stained with an Osmium Thiocarbohydrazide Osmium protocol using Uranyl Acetate en bloc staining (Deerinck et al., 2010). Scale bar: 10 μm. (B) Mouse brain tissue stained as panel A, replacing uranyl acetate with lanthanide salts. Scale bar: 10 μm. (C) Arabidopsis thaliana root tip that was en bloc stained with uranyl acetate. Scale bar: 5 μm. (D) Arabidopsis root tip done under same conditions as panel C, except replacing uranyl acetate with lanthanide salts. Scale bar : 5 μm. (E) Mouse brain tissue in which contrast staining and embedding was performed using a microwave oven. imaged in a Zeiss Merlin with a Gatan Digiscan II detector. Scale bar: 1 μm. (F) A similar sample to panel E was imaged in a Zeiss Auriga using a BSE detector. Scale bar: 1 μm.
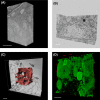
Consecutive SBF-SEM and FIB-SEM imaging. (A) A volume of 26,75 × 38,20 × 12,83 μm of normal mouse skin reconsted from SBF-SEM imaging. Undifferentiated basal layer (*) and differentiated layers (**granular layer and *** cornified layer) can be distinguished. Scale Bar: 10 μm. (B) A similar sample was used for imaging in a FIB-SEM. A basal layer keratinocyte is shown where subcellular organelles and connections between cells, such as desmosomes, are visible. Scale Bar: 1 μm. (C) Medicago truncatula root tips imaged by SBF-SEM. Orthogonal views of a volume of 177 × 166 × 60 μm consisting of 600, 100 nm slices is shown. Segmentation in red shows the cell walls and gives an overview on the general organization of the cells within the root tip. Scale Bar: 20 μm. (D) The same sample was transferred to a Zeiss Auriga FIB-SEM at 5 nm isotropic voxels. A selected region of 8 × 8 × 3 μm is shown in a 3D representation showing mitochondria, the Golgi apparatus, and plasmodesmata connecting to the ER. Scale Bar: 1 μm.
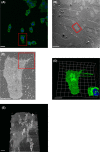
CLEM. (A) Human lung epithelial A549 cells were grown on gridded coverslips and imaged by confocal microscopy, with wheat germ agglutinin in green and nuclear dye in blue the cells of interest are highlighted in the red box. Scale bar: 24 μm. (B) An SEM image, using SE2 detector shows the lines of the coverslip grids. Scale bar: 50 μm. (C) An image of the block-face was taken at 15 kV, using a BSE detector confirming the identification of the cell of interest. The red box indicates the area that will be imaged with FIB-SEM. (D) 3D reconstruction of a confocal z-stack, showing the cell of interest. Scale bar: 10 μm. (E) 3D reconstruction of the FIB-SEM image of the area indicated with a red box in panel C. The imaged volume dimensions are 16,92 × 11,47 × 9,58 μm. Fiji was used for image processing. Scale bar: 20 μm.
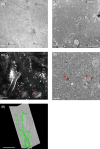
CLEM. (A) NIRB was used to introduce scars in brain tissue, after live-cell imaging, in order to create reference points that allow correlation of the LM images to SBF-SEM data. A bright-field image shows the presence of four laser-brands (1–4). Scale bar: 20 μm. (B) The first image of a stack, generated by SBF-SEM, shows laser-brands 2 and 4 in the corners. Importantly, no apparent damage to the ultra-structure surrounding the brands is visible. Scale bar: 10 μm. (C) A confocal image showing in vivo imaging of astrocyte Ca2+ activity. Red arrows indicate two areas of astrocyte activity a few microns below the laser-brands. Scale bar: 30 μm. (D) Based on the laser-brands, the two astrocytes, indicated in panel C, could be retraced in the SBF-SEM data-set. Arrowheads indicate the astrocytes in a single SEM image. Scale bar: 10 μm. (E) Manual segmentation of the astrocytes was done using IMOD. 2 orthogonal views and the segmented astrocyte in green are shown in the volume. Scale bar: 10 μm.
Similar articles
-
Mourik MJ, Faas FGA, Zimmermann H, Eikenboom J, Koster AJ. Mourik MJ, et al. J Microsc. 2015 Aug;259(2):97-104. doi: 10.1111/jmi.12222. Epub 2015 Jan 23. J Microsc. 2015. PMID: 25644989 Free PMC article.
-
Volume scanning electron microscopy for imaging biological ultrastructure.
Titze B, Genoud C. Titze B, et al. Biol Cell. 2016 Nov;108(11):307-323. doi: 10.1111/boc.201600024. Epub 2016 Aug 12. Biol Cell. 2016. PMID: 27432264 Review.
-
Three-dimensional imaging of adherent cells using FIB/SEM and STEM.
Villinger C, Schauflinger M, Gregorius H, Kranz C, Höhn K, Nafeey S, Walther P. Villinger C, et al. Methods Mol Biol. 2014;1117:617-38. doi: 10.1007/978-1-62703-776-1_27. Methods Mol Biol. 2014. PMID: 24357382
-
A workflow for 3D-CLEM investigating liver tissue.
Kremer A, VAN Hamme E, Bonnardel J, Borghgraef P, GuÉrin CJ, Guilliams M, Lippens S. Kremer A, et al. J Microsc. 2021 Mar;281(3):231-242. doi: 10.1111/jmi.12967. Epub 2020 Oct 27. J Microsc. 2021. PMID: 33034376
-
New developments in electron microscopy for serial image acquisition of neuronal profiles.
Kubota Y. Kubota Y. Microscopy (Oxf). 2015 Feb;64(1):27-36. doi: 10.1093/jmicro/dfu111. Epub 2015 Jan 5. Microscopy (Oxf). 2015. PMID: 25564566 Review.
Cited by
-
Ravi RT, Leung MR, Zeev-Ben-Mordehai T. Ravi RT, et al. Open Biol. 2020 Sep;10(9):200186. doi: 10.1098/rsob.200186. Epub 2020 Sep 16. Open Biol. 2020. PMID: 32931719 Free PMC article. Review.
-
Composition, structure and function of the corneal stroma.
Espana EM, Birk DE. Espana EM, et al. Exp Eye Res. 2020 Sep;198:108137. doi: 10.1016/j.exer.2020.108137. Epub 2020 Jul 11. Exp Eye Res. 2020. PMID: 32663498 Free PMC article. Review.
-
Goggin P, Ho EML, Gnaegi H, Searle S, Oreffo ROC, Schneider P. Goggin P, et al. Bone. 2020 Feb;131:115107. doi: 10.1016/j.bone.2019.115107. Epub 2019 Oct 24. Bone. 2020. PMID: 31669251 Free PMC article. Review.
-
McBride EL, Rao A, Zhang G, Hoyne JD, Calco GN, Kuo BC, He Q, Prince AA, Pokrovskaya ID, Storrie B, Sousa AA, Aronova MA, Leapman RD. McBride EL, et al. J Struct Biol. 2018 Jun;202(3):216-228. doi: 10.1016/j.jsb.2018.01.012. Epub 2018 Feb 1. J Struct Biol. 2018. PMID: 29408702 Free PMC article.
-
Nakao A, Miyazaki N, Ohira K, Hagihara H, Takagi T, Usuda N, Ishii S, Murata K, Miyakawa T. Nakao A, et al. Mol Brain. 2017 Dec 12;10(1):60. doi: 10.1186/s13041-017-0339-2. Mol Brain. 2017. PMID: 29233179 Free PMC article.
References
-
- Abandowitz HM. Geissinger HD. Preparation of cells from suspensions for correlative scanning electron and interference microscopy. Histochemistry. 1975;45:89–94. - PubMed
-
- Abbe E. Beiträge zur Theorie des Mikroskops und der mikroskopischen Wahrnehmung. Archiv für Mikroskopische Anatomie. 1873;9:413–468.
-
- Ballerini M, Milani M, Batani M. Squadrini F. Focused ion beam techniques for the analysis of biological samples: a revolution in ultramicroscopy. Proc. SPIE. 2001;4261:92–104.
-
- Bishop D, Nikic I, Brinkoetter M, Knecht S, Potz S, Kerschensteiner M. Misgeld T. Near-infrared branding efficiently correlates light and electron microscopy. Nat. Meth. 2011;8:568–570. - PubMed
Publication types
MeSH terms
LinkOut - more resources
Full Text Sources
Other Literature Sources