Extreme water loss and abiotic O2 buildup on planets throughout the habitable zones of M dwarfs - PubMed
- ️Invalid Date
Extreme water loss and abiotic O2 buildup on planets throughout the habitable zones of M dwarfs
R Luger et al. Astrobiology. 2015 Feb.
Abstract
We show that terrestrial planets in the habitable zones of M dwarfs older than ∼1 Gyr could have been in runaway greenhouses for several hundred million years following their formation due to the star's extended pre-main sequence phase, provided they form with abundant surface water. Such prolonged runaway greenhouses can lead to planetary evolution divergent from that of Earth. During this early runaway phase, photolysis of water vapor and hydrogen/oxygen escape to space can lead to the loss of several Earth oceans of water from planets throughout the habitable zone, regardless of whether the escape is energy-limited or diffusion-limited. We find that the amount of water lost scales with the planet mass, since the diffusion-limited hydrogen escape flux is proportional to the planet surface gravity. In addition to undergoing potential desiccation, planets with inefficient oxygen sinks at the surface may build up hundreds to thousands of bar of abiotically produced O2, resulting in potential false positives for life. The amount of O2 that builds up also scales with the planet mass; we find that O2 builds up at a constant rate that is controlled by diffusion: ∼5 bar/Myr on Earth-mass planets and up to ∼25 bar/Myr on super-Earths. As a result, some recently discovered super-Earths in the habitable zone such as GJ 667Cc could have built up as many as 2000 bar of O2 due to the loss of up to 10 Earth oceans of water. The fate of a given planet strongly depends on the extreme ultraviolet flux, the duration of the runaway regime, the initial water content, and the rate at which oxygen is absorbed by the surface. In general, we find that the initial phase of high luminosity may compromise the habitability of many terrestrial planets orbiting low-mass stars.
Figures
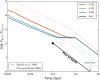
Evolution of the XUV flux received by planets close to the inner edge of the HZ orbiting 0.1, 0.2, and 0.3 M⊙ M dwarfs, scaled to that received by present Earth, ⊕=4.64 erg/cm2/s. Solid lines correspond to the model adopted in this paper, where fluxes are calculated using the stellar evolution model of Baraffe et al. (1998) and the XUV evolution of Ribas et al. (2005), assuming a saturation time tsat of 1 Gyr and a saturation fraction of 10−3. Dashed lines correspond to the empirical model of Penz and Micela (2008), who assume a single XUV luminosity for all M dwarfs; see text for a discussion. The evolution of the flux at Earth is shown for reference as a black line. The dot corresponds to the earliest time for which the study of Ribas et al. (2005) has data for solar-type stars and is approximately equal to the saturation time for the Sun; it is also roughly the formation time for Earth.
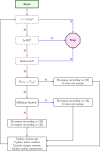
Flowchart representing a single integration of our code. The three halting conditions are represented by dashed boxes. See Section 3 for a detailed description of our model.
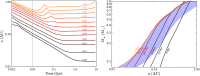
Evolution of the position of the inner edge of the empirical HZ (RV limit) as a function of time. Left: Location of the RV limit versus stellar age for stars between 0.08 M⊙ and 1 M⊙. The vertical dashed line corresponds to the 10 Myr formation time assumed in our model. Right: Contours of the RV limit on a classical HZ plot between 3 Myr (black line) and 1 Gyr (orange line). The empirical HZ at 1 Gyr is shaded in blue for reference. Note that the inner edge moves in by about an order of magnitude for the lowest-mass M dwarfs.
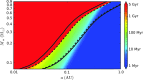
Duration of the runaway greenhouse for planets that formed at 10 Myr with abundant surface water. The solid black lines correspond to the RV (left) and EM (right) limits (the empirical HZ); the dashed lines correspond to the RG (left) and MG (right) limits (the theoretical HZ). All limits are those at a stellar age of 5 Gyr. Red corresponds to planets that never leave the runaway state, since these are always interior to the HZ. Blue corresponds to planets that spend less than 1 Myr in a runaway greenhouse. Planets throughout the HZs of all M dwarfs spend a substantial amount of time in a runaway greenhouse.
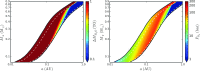
Energy-Limited Escape: 1 M⊕, 1 TO. Total amount of water lost and amount of oxygen absorbed at the surface for a 1 M⊕ planet formed at 10 Myr with 1 TO of surface water, assuming the planet is in a runaway interior to the RG limit, the oxygen is instantaneously absorbed by the surface, and the escape is energy-limited. The solid lines are the empirical HZ bounds; the dashed lines are the theoretical HZ bounds. See Fig. 6 for more details.
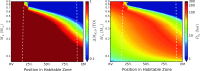
Energy-Limited Escape: 1 M⊕, 1 TO. Same as Fig. 5 (1 TO, energy-limited) but in an expanded view of the HZ. The axes correspond to the stellar mass (vertical) and the position of the planet within the HZ at 5 Gyr (horizontal). The “position in habitable zone” is the fractional distance between the RV limit and the EM limit (the empirical HZ). The dashed lines once again represent the RG and MG limits. Left: Total water lost in TO after 5 Gyr. Dark blue corresponds to less than 0.1 TO; dark red corresponds to complete desiccation. Most planets in the HZ of M dwarfs are completely desiccated; conversely, those close to the outer edge of high-mass M dwarfs and throughout most of the HZ of K dwarfs lose little or no water. Interior to the RG limit, planets around stars of all masses are completely desiccated. Right: Total amount of oxygen absorbed by the surface in bar. Dark blue corresponds to insignificant O2 buildup; dark red corresponds to 200 bar of oxygen. Planets that lose significant amounts of water also undergo extreme surface oxidation.
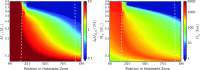
Energy-Limited Escape: 1 M⊕, 10 TO. Same as Fig. 6 (energy-limited escape) but for an initial water content of 10 TO. Note the change in the color-bar scales. Planets throughout most of the HZ of M dwarfs now lose at least 1 TO of water; those close to the RG limit and around low-mass M dwarfs lose close to 10 TO. Most planets now retain several hundred to ∼1000 bar of O2.
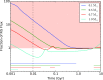
Evolution of the flux received by planets that are at the inner edge of the theoretical HZ at 5 Gyr for different stellar masses (blue: 0.1 M⊙, green: 0.5 M⊙, red: 0.7 M⊙, cyan: 1.0 M⊙). The vertical axis is the bolometric flux normalized to the runaway greenhouse flux; the region shaded in pink corresponds to planets that are interior to the RG limit. The dashed vertical line corresponds to the 10 Myr formation time we assume in our model. The horizontal lines at the bottom of the plot indicate the times during which planets are in a runaway greenhouse. Planets around late-type M dwarfs experience a single long runaway prior to the star's arrival on the MS. Planets around G dwarfs experience an initial runaway phase if they form in the first ∼2 Myr as well as a late phase due to the star's brightening during the MS. Planets around K dwarfs (0.6 M⊙≲M★≲0.9 M⊙) experience three runaway episodes, due to the temporary increase in the star's luminosity prior to the arrival on the MS between ∼50 and ∼100 Myr. This is due to the fact that these stars switch from convective to radiative energy transport at the end of their Hayashi contraction phase. See text for a discussion.
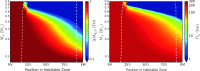
Diffusion-Limited Escape, 1 M⊕, 1 TO. Similar to Fig. 6 (1 TO) but assuming that the escape of hydrogen is diffusion-limited. This corresponds to planets with slow/ineffective oxygen sinks that retain all the photolytically produced O2 in their atmospheres. While water loss amounts are generally lower than in the energy-limited case, planets throughout a large fraction of the HZ of M dwarfs are still desiccated. Moreover, the amount of oxygen that builds up is substantially greater than in Fig. 6, since the oxygen cannot escape if the loss of hydrogen is diffusion-limited. Thus, planets that lose 1 TO of water build up ×270=240 bar of O2 in their atmospheres.
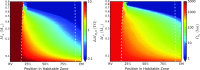
Diffusion-Limited Escape: 1 M⊕, 10 TO. Same as the previous figure (diffusion-limited escape) but for an initial water content of 10 TO; compare to Fig. 7, the corresponding energy-limited case. Once again, water loss amounts are smaller, but oxygen amounts greater than 2000 bar are now possible around the lowest-mass M dwarfs.
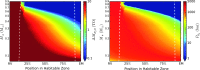
Energy-Limited Escape: 5 M⊕, 10 TO. Similar to the previous figures but for a super-Earth of mass 5 M⊕ with 10 TO, assuming energy-limited escape. Note the large fraction of the HZ in the lower left portion of the left panel where planets are completely desiccated. Elsewhere, super-Earths lose several TO of water. In the right panel, thousands of bar of O2 are absorbed at the surface of planets throughout most of the HZ of M dwarfs.
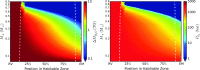
Diffusion-Limited Escape: 5 M⊕, 10 TO. Similar to Fig. 11 (a 5 M⊕ super-Earth with 10 TO of surface water) but assuming diffusion-limited escape. Despite a slight decrease in the total water loss amounts, the results are very similar to those in the energy-limited case. In general, these planets lose several TO of water and build up several hundred to a few thousand bar of O2 in their atmospheres.
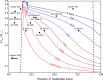
A selection of M and K dwarf super-Earths that could have detectable O2 atmospheres if they formed with abundant surface water; as before, the dashed lines represent the RG and MG limits. Calculations were performed assuming a mass of 5 M⊕, an initial water content of 10 TO, and diffusion-limited escape. Contours correspond to the equivalent O2 atmospheric pressure in bar at the end of the runaway phase, assuming all the O2 remains in the atmosphere. Of the planets shown here, only Kepler-62f does not build up any oxygen.
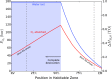
Cross section along M★=0.4 M⊙ in Fig. 6, showing the oxygen absorbed by the surface (red) and total amount of water lost (blue) as a function of the position in the HZ. Water loss scales with the time spent in the runaway phase; interior to a critical distance, complete desiccation occurs (shaded region). From right to left, the O2 amount initially increases due to the increase in the amount of water lost. However, interior to the critical distance, complete desiccation occurs at progressively earlier times. The higher XUV flux early on results in more oxygen escape and less buildup. See text for a discussion.
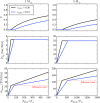
Dependence of the oxygen escape parameter η (top), the rate of oxygen buildup (center), and the ocean loss rate
ocean (bottom) on the XUV flux for a 1 M⊕ Earth (left) and a 5 M⊕ super-Earth (right) in the energy-limited regime. Results for two different XUV efficiencies are plotted: 0.30 (black) and 0.15 (blue). For η=0, corresponding to
XUV<
crit, the rates of oxygen buildup and ocean loss are linear in
XUV. For η>0, the oxygen buildup rate is constant at ∼5 bar/Myr for the Earth and ∼25 bar/Myr for the super-Earth. The rate of ocean loss is still linear in
XUV but increases more slowly. For reference, in the bottom panel we plot the diffusion-limited ocean loss rate as a dashed red line.
Similar articles
-
Luger R, Barnes R, Lopez E, Fortney J, Jackson B, Meadows V. Luger R, et al. Astrobiology. 2015 Jan;15(1):57-88. doi: 10.1089/ast.2014.1215. Astrobiology. 2015. PMID: 25590532
-
Habitable zones around main sequence stars.
Kasting JF, Whitmire DP, Reynolds RT. Kasting JF, et al. Icarus. 1993 Jan;101(1):108-28. doi: 10.1006/icar.1993.1010. Icarus. 1993. PMID: 11536936
-
M stars as targets for terrestrial exoplanet searches and biosignature detection.
Scalo J, Kaltenegger L, Segura A, Fridlund M, Ribas I, Kulikov YN, Grenfell JL, Rauer H, Odert P, Leitzinger M, Selsis F, Khodachenko ML, Eiroa C, Kasting J, Lammer H. Scalo J, et al. Astrobiology. 2007 Feb;7(1):85-166. doi: 10.1089/ast.2006.0125. Astrobiology. 2007. PMID: 17407405 Review.
-
Gebauer S, Grenfell JL, Lehmann R, Rauer H. Gebauer S, et al. Astrobiology. 2018 Jul;18(7):856-872. doi: 10.1089/ast.2017.1723. Astrobiology. 2018. PMID: 30035637
-
A reappraisal of the habitability of planets around M dwarf stars.
Tarter JC, Backus PR, Mancinelli RL, Aurnou JM, Backman DE, Basri GS, Boss AP, Clarke A, Deming D, Doyle LR, Feigelson ED, Freund F, Grinspoon DH, Haberle RM, Hauck SA 2nd, Heath MJ, Henry TJ, Hollingsworth JL, Joshi MM, Kilston S, Liu MC, Meikle E, Reid IN, Rothschild LJ, Scalo J, Segura A, Tang CM, Tiedje JM, Turnbull MC, Walkowicz LM, Weber AL, Young RE. Tarter JC, et al. Astrobiology. 2007 Feb;7(1):30-65. doi: 10.1089/ast.2006.0124. Astrobiology. 2007. PMID: 17407403 Review.
Cited by
-
Mantle redox state drives outgassing chemistry and atmospheric composition of rocky planets.
Ortenzi G, Noack L, Sohl F, Guimond CM, Grenfell JL, Dorn C, Schmidt JM, Vulpius S, Katyal N, Kitzmann D, Rauer H. Ortenzi G, et al. Sci Rep. 2020 Jul 2;10(1):10907. doi: 10.1038/s41598-020-67751-7. Sci Rep. 2020. PMID: 32616773 Free PMC article.
-
Lehmer OR, Catling DC. Lehmer OR, et al. Astrophys J. 2017 Aug 20;845(2):130. doi: 10.3847/1538-4357/aa8137. Epub 2017 Aug 18. Astrophys J. 2017. PMID: 33041344 Free PMC article.
-
Exoplanet Biosignatures: A Review of Remotely Detectable Signs of Life.
Schwieterman EW, Kiang NY, Parenteau MN, Harman CE, DasSarma S, Fisher TM, Arney GN, Hartnett HE, Reinhard CT, Olson SL, Meadows VS, Cockell CS, Walker SI, Grenfell JL, Hegde S, Rugheimer S, Hu R, Lyons TW. Schwieterman EW, et al. Astrobiology. 2018 Jun;18(6):663-708. doi: 10.1089/ast.2017.1729. Epub 2018 May 4. Astrobiology. 2018. PMID: 29727196 Free PMC article. Review.
-
Atmospheric Beacons of Life from Exoplanets Around G and K Stars.
Airapetian VS, Jackman CH, Mlynczak M, Danchi W, Hunt L. Airapetian VS, et al. Sci Rep. 2017 Nov 2;7(1):14141. doi: 10.1038/s41598-017-14192-4. Sci Rep. 2017. PMID: 29097693 Free PMC article.
-
Super-Earths, M Dwarfs, and Photosynthetic Organisms: Habitability in the Lab.
Claudi R, Alei E, Battistuzzi M, Cocola L, Erculiani MS, Pozzer AC, Salasnich B, Simionato D, Squicciarini V, Poletto L, La Rocca N. Claudi R, et al. Life (Basel). 2020 Dec 24;11(1):10. doi: 10.3390/life11010010. Life (Basel). 2020. PMID: 33374408 Free PMC article.
References
-
- Abe Y., Abe-Ouchi A., Sleep N.H., and Zahnle K.J. (2011) Habitable zone limits for dry planets. Astrobiology 11:443–460 - PubMed
-
- Anbar A.D., Duan Y., Lyons T.W., Arnold G.L., Kendall B., Creaser R.A., Kaufman A.J., Gordon G.W., Scott C., Garvin J., and Buick R. (2007) A whiff of oxygen before the Great Oxidation Event? Science 317:1903–1906 - PubMed
-
- Anglada-Escudé G., Tuomi M., Gerlach E., Barnes R., Heller R., Jenkins J.S., Wende S., Vogt S.S., Butler R.P., Reiners A., and Jones H.R.A. (2013) A dynamically packed planetary system around GJ 667C with three super-Earths in its habitable zone. Astron Astrophys 556:A126
-
- Ballard S., Charbonneau D., Fressin F., Torres G., Irwin J., Desert J.-M., Newton E., Mann A.W., Ciardi D.R., Crepp J.R., Henze C.E., Bryson S.T., Howell S.B., Horch E.P., Everett M.E., and Shporer A. (2013) Exoplanet characterization by proxy: a transiting 2.15 R⊕ planet near the habitable zone of the late K dwarf Kepler-61. Astrophys J 773, doi:10.1088/0004-637X/773/2/98 - DOI
-
- Baraffe I., Chabrier G., Allard F., and Hauschildt P.H. (1998) Evolutionary models for solar metallicity low-mass stars: mass-magnitude relationships and color-magnitude diagrams. Astron Astrophys 337:403–412
Publication types
MeSH terms
Substances
LinkOut - more resources
Full Text Sources
Other Literature Sources
Research Materials