Identification of a spinal circuit for light touch and fine motor control - PubMed
- ️Thu Jan 01 2015
Identification of a spinal circuit for light touch and fine motor control
Steeve Bourane et al. Cell. 2015.
Abstract
Sensory circuits in the dorsal spinal cord integrate and transmit multiple cutaneous sensory modalities including the sense of light touch. Here, we identify a population of excitatory interneurons (INs) in the dorsal horn that are important for transmitting innocuous light touch sensation. These neurons express the ROR alpha (RORα) nuclear orphan receptor and are selectively innervated by cutaneous low threshold mechanoreceptors (LTMs). Targeted removal of RORα INs in the dorsal spinal cord leads to a marked reduction in behavioral responsiveness to light touch without affecting responses to noxious and itch stimuli. RORα IN-deficient mice also display a selective deficit in corrective foot movements. This phenotype, together with our demonstration that the RORα INs are innervated by corticospinal and vestibulospinal projection neurons, argues that the RORα INs direct corrective reflex movements by integrating touch information with descending motor commands from the cortex and cerebellum.
Copyright © 2015 Elsevier Inc. All rights reserved.
Figures
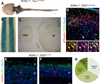
(A–C) Images from P10 RORαCre; TauLSL-nlslacZ mice showing β-galactosidase expression in the central nervous system (A) and spinal cord (B and C). C shows transverse section through the spinal cord. (D) Section through P10 RORαCre; R26LSL-tdTomato lumbar spinal cord stained with antibodies to RORα (green) and NeuN (blue). tdTomato+ fluorescence (red) was visualized without staining. Merged image shows tdTomato is largely restricted to RORα+/NeuN+ neurons in lamina IIi/III. The tdTomato+ cells located outside of lamina II/III do not express NeuN. Arrows indicate double-labeled neurons. Arrowhead in D indicates a tdTomato+ blood vessel. Asterisk marks a tdTomato+ glial cell. (E–F) mCherry-labeled neurons (red) in the lumbar cord of P21 RORαCre; R26LSL-TVA mice. Their location in relation to excitatory PKCγ+ neurons (green) and vGluT1 sensory afferents (blue) is shown. (G) Summary of the morphological profile of 51 RORα INs. Scale bars: 1000 µm (A), 500 µm (B), 200 µm (C), 100 µm (D), 25 µm (E and F). See also Figure S1.
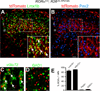
(A–B) Transverse sections through P10 RORαCre; R26LSL-tdTomato lumbar dorsal spinal cord showingRORα os coexpressed with Lmx1b, but not with Pax2. (C–D) Sections from P10 RORαCre; R26LSL-tdTomato; GAD1-GFP spinal cord showing tdTomato is coexpressed with vGluT2 mRNA (C, green), but not GFP (D, green). (E) Quantification of dorsal spinal cord marker expression in RORα INs. Cell counts obtained from 3 spinal cords were pooled and expressed as mean ± SEM. Cell counts for RORα INs expressing Lmx1b (940/1093), vGluT2 (904/987), Pax2 (15/1197) and GAD1 (22/845). Scale bars: 100 µm (A–B), 10 µm (C–D). See also Figure S2
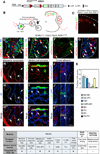
(A–B) Schematic showing the R26ds-HTB allele and strategy used for tracing monosynaptic inputs to the RORα INs using an EnvA-pseudotyped ΔG-mCherry-rabies virus. (C) Section through P10 RORαCre; Cdx2::FlpO; R26ds-HTB spinal cord showing mCherry-rabies virus labbled cells and RORα INs (green). mCherry+/GFP− cells represent transynaptically-labeled presynaptic neurons. (D–G) Sections from P10 RORαCre; Cdx2::FlpO; R26ds-HTB lumbar DRG showing presynaptically labeled sensory neurons and sensory neuron subtype markers as indicated (H) Section of hindlimb footpad stained with antibodies to mCherry (red) and NF (blue) showing Meissner corpuscles innervation. (I) Section of hindlimb hairy skin stained with antibodies to mCherry (red), NF (blue) and TrpV3 (green), which recognizes Merkel cells. (J) Section of hairy skin showing a D-hair afferent following staining with antibodies to mCherry (red), TrkB (green) and DAPI (blue) (K) Quantification of sensory neuronal markers in relation to total number of mCherry+ neurons, n = 3 mice. (L) Summary of sensory afferent types that are presynaptic to the RORα INs. Abbreviations: NF, neurofilament; CB, calbindin; CR, calretinin; TH, Tyrosine Hydroxylase. Dashed lines define the boundary of the DRG (D–G) and hair follicles (J). Arrows indicate double-labeled neurons or sensory afferents. Scale bar: 50 µm (D–G), 25 µm (H–J). See also Figure S3.
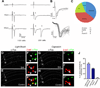
(A) Example of a RORα IN in L4 displaying an A fiber monosynaptic current triggered by low intensity stimulus. Monosynaptic connectivity was confirmed by a 2Hz frequency stimulation. (B) Example of a RORα IN with dual A and C inputs. (C) Classification of potentials in RORα INs induced by dorsal root stimulation. n = 31 cells. (D–I) Sections through the lumbar spinal cord of P6 RORαCre; R26ds-HTB mice stained with antibodies to c-Fos and β-gal after light brushing (D–F) or injecting capsaicin into the footpad (G–I). Arrowheads in F and I indicate c-Fos+ RORα INs. Scale bars: 50 µm. Panels E and H show the contralateral side. (J) Fraction of RORα INs (β-gal+) coexpressing c-Fos. Only lamina IIi/III cells located within the domain displaying elevated c-Fos expression were counted. Cell counts of RORα INs exhibiting c-Fos immunoreactivity: brush stimulation of glabrous skin, 1593/2830 RORα cells; brush stimulation of hairy skin, 834/2103 RORα cells; capsaicin injection, 20/711 RORα cells. n = 4 animals for each experiment. Data: mean ± SEM. Scale bar: 100 µm (D,E,G,H).
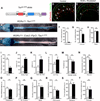
(A) Schematic illustrating the Tauds-DTR allele. (B–C) Brain and spinal cords from P84 RORαCre; Tauds-DTR (control) and RORαCre; Cdx2::FlpO; Tauds-DTR (RORα IN-ablated) mice showing β-galactosidase reporter expression. (D–E) Transverse sections through the lumbar dorsal horn of adult control (D) and RORα IN-ablated (E) mice 14 days after injecting DTX showing tdTomato (red) and Lmx1b (green) expression. Arrows indicate double-labeled neurons. (F) RORα+ IN numbers are reduced by 95% following DTX-ablation (3.16±0.46 cells versus 70.30±6.01 cells), *** p<0.001. (G) Lmx1b-expressing cells are reduced by 30% in RORα IN-ablated cords (112.1±6.9 cells versus 161.0±9.5 cells), * p <0.05. (H) Pax2+ cell numbers are unchanged in RORα IN-ablated cords (113.1±4.7 cells versus 113.9±2.9 cells), p >0.05. (I) RORα IN-ablated mice show a significant decrease in paw withdrawal to dynamic light brush (30±7.9% versus 76±6.5% of trials, *** p <0.001). (J–K) RORα IN-ablated mice show a significant increase in latency) to static light touch as measured using the sticky tape test (J, 192.9±20.4 secs versus 78.9 ± 17.7 secs, *** p <0.001) and detecting a 1.5mm alligator clip on the hairy skin (K, 47.3 ± 11.5 to 20.9 ± 4.2, * p < 0.05). (L–N) Mechanical pain measured by von Frey filament, Randall Selitto and pinprick does not differ between control and RORα IN-ablated mice. (O–R) Sensitivity to heat (Hargreaves) and cooling (acetone) in RORα IN-ablated mice is normal, as are responses to 48/80 and chloroquine. (S–T) Chemical pain induced by injecting capsaicin or formalin into the footpad is also unchanged in RORα IN-ablated mice. Data: mean ± SEM. ns, p values above 0.05 are not significant. n = number of mice tested. Scale Bar: 100 µm. See also Figure S4.
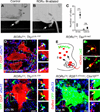
(A–B) Images showing control (A) and RORα IN-ablated (B) mice crossing a 5mm wide beam. Arrow indicates position of the foot. Note the position of the foot in B showing a foot slip (C) Number of slips and footfalls for 5 mm and 12 mm wide beams. White circles indicate control mice (17 and 11 mice tested for 5 and 12 mm beams, respectively). Black circles indicate RORα IN-ablated mice (19 and 12 mice tested for 5 and 12 mm beams, respectively). (D and H) P21 RORαCre; Thy1LSL-YFP spinal cord sections stained with antibodies to GFP (green), vGluT2 (blue) showing synaptic boutons (arrows) on ChAT+ motor neurons (D), and ChAT+ V0c neurons (H). (E–G) Retrograde monosynaptic mCherry-rabies virus labeling of RORα INs following injection into the tibialis anterior (F) and gastrocnemius muscles (G). The tracing protocol is shown in E. (I) P21 RORαCre; R26LSL-tdTomato; Chx10CFP spinal cord section stained with antibodies to DsRed (red); GFP (green) and vGluT2 (blue), showing synaptic boutons (arrows) on a Chx10+ V2a IN (green). Data: mean ± SEM, ** p <0.01, ns, p> 0.05 Scale bars: 5 µm (D), 10 µm (F–G), 2 µm (H,I). See also Figure S5.
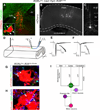
(A–C) Transverse sections from a P15 RORαCre; Cdx2::FlpO; R26ds-HTB brain 5 days after infecting RORα INs in the lumbar cord with an EnvA G-deleted rabies–mCherry virus. Transsynaptically labeled neurons (red) in the lateral vestibular nucleus (LVN) are innervated by calbindin+ Purkinje cells afferents (green, A). Pyramidal neurons in the frontal motor cortex area are transsynaptically labeled (B and C). (D) Hemicord preparation showing the stimulation-whole patch recording set-up for RORα INs. The region of the dorsal funiculus that contains corticospinal axons was stimulated with a concentric bipolar electrode 5 segments (3–4 mm) rostral to the recorded cell (i). A single dorsal root (L4-L6) was stimulated with a glass suction electrode (ii). RORα cells (green) in L4 were patched and EPSCs were recorded (iii). R, rostral; C, caudal; D, dorsal; V, ventral. (E) Representative recording of an A fiber-type dorsal root evoked potential in a RORα IN. Magnification of onset is shown. (F) Putative corticospinal tract evoked EPSCs in the RORα IN that is shown in E. Latency and jitter properties are consistent with monosynaptic connectivity (see magnification of onset). (G) Section from a P21 RORαCre; R26LSL-tdTomato spinal cord showing PKCγ+/vGluT1+ corticospinal processes contacting a RORα IN (red). Arrow indicates a PKCγ+/vGluT1+ corticospinal-derived synaptic bouton. Arrowhead indicates a vGluT1+ sensory contact. (H) Section from a P21 RORαCre; R26LSL-tdTomato; Emx1GFP spinal cord stained with antibodies to DsRed (red), GFP (green) and vGluT1 (blue), which confirms GFP+/vGluT1+ corticospinal contacts (arrows) onto RORα INs (red). Arrowhead indicates a vGluT1+ sensory contact. (I) Schematic showing the RORα IN connectivity and an outline of the RORα IN spinal circuit for light touch. NB. Arrows to and from the cerebellum do not indicate direct connections. Scale bar: 2 µm (G,H). See also Figure S6.
Similar articles
-
Paixão S, Loschek L, Gaitanos L, Alcalà Morales P, Goulding M, Klein R. Paixão S, et al. Neuron. 2019 Nov 20;104(4):749-764.e6. doi: 10.1016/j.neuron.2019.08.029. Epub 2019 Oct 2. Neuron. 2019. PMID: 31586516 Free PMC article.
-
Contribution of dorsal horn CGRP-expressing interneurons to mechanical sensitivity.
Löken LS, Braz JM, Etlin A, Sadeghi M, Bernstein M, Jewell M, Steyert M, Kuhn J, Hamel K, Llewellyn-Smith IJ, Basbaum A. Löken LS, et al. Elife. 2021 Jun 1;10:e59751. doi: 10.7554/eLife.59751. Elife. 2021. PMID: 34061020 Free PMC article.
-
Touch and tactile neuropathic pain sensitivity are set by corticospinal projections.
Liu Y, Latremoliere A, Li X, Zhang Z, Chen M, Wang X, Fang C, Zhu J, Alexandre C, Gao Z, Chen B, Ding X, Zhou JY, Zhang Y, Chen C, Wang KH, Woolf CJ, He Z. Liu Y, et al. Nature. 2018 Sep;561(7724):547-550. doi: 10.1038/s41586-018-0515-2. Epub 2018 Sep 12. Nature. 2018. PMID: 30209395 Free PMC article.
-
PKCγ interneurons, a gateway to pathological pain in the dorsal horn.
Artola A, Voisin D, Dallel R. Artola A, et al. J Neural Transm (Vienna). 2020 Apr;127(4):527-540. doi: 10.1007/s00702-020-02162-6. Epub 2020 Feb 27. J Neural Transm (Vienna). 2020. PMID: 32108249 Review.
-
Uncovering the Cells and Circuits of Touch in Normal and Pathological Settings.
Moehring F, Halder P, Seal RP, Stucky CL. Moehring F, et al. Neuron. 2018 Oct 24;100(2):349-360. doi: 10.1016/j.neuron.2018.10.019. Neuron. 2018. PMID: 30359601 Free PMC article. Review.
Cited by
-
Neuronal diversity in the somatosensory system: bridging the gap between cell type and function.
Gatto G, Smith KM, Ross SE, Goulding M. Gatto G, et al. Curr Opin Neurobiol. 2019 Jun;56:167-174. doi: 10.1016/j.conb.2019.03.002. Epub 2019 Apr 4. Curr Opin Neurobiol. 2019. PMID: 30953870 Free PMC article. Review.
-
Sathyamurthy A, Johnson KR, Matson KJE, Dobrott CI, Li L, Ryba AR, Bergman TB, Kelly MC, Kelley MW, Levine AJ. Sathyamurthy A, et al. Cell Rep. 2018 Feb 20;22(8):2216-2225. doi: 10.1016/j.celrep.2018.02.003. Cell Rep. 2018. PMID: 29466745 Free PMC article.
-
Haenraets K, Albisetti GW, Foster E, Wildner H. Haenraets K, et al. J Vis Exp. 2018 May 12;(135):57382. doi: 10.3791/57382. J Vis Exp. 2018. PMID: 29806830 Free PMC article.
-
Spinal Circuits for Touch, Pain, and Itch.
Koch SC, Acton D, Goulding M. Koch SC, et al. Annu Rev Physiol. 2018 Feb 10;80:189-217. doi: 10.1146/annurev-physiol-022516-034303. Epub 2017 Sep 27. Annu Rev Physiol. 2018. PMID: 28961064 Free PMC article. Review.
-
Identification of Early RET+ Deep Dorsal Spinal Cord Interneurons in Gating Pain.
Cui L, Miao X, Liang L, Abdus-Saboor I, Olson W, Fleming MS, Ma M, Tao YX, Luo W. Cui L, et al. Neuron. 2016 Sep 7;91(5):1137-1153. doi: 10.1016/j.neuron.2016.07.038. Epub 2016 Aug 18. Neuron. 2016. PMID: 27545714 Free PMC article.
References
Publication types
MeSH terms
Substances
Grants and funding
LinkOut - more resources
Full Text Sources
Other Literature Sources
Molecular Biology Databases
Research Materials