Therapeutic genome editing: prospects and challenges - PubMed
Review
Therapeutic genome editing: prospects and challenges
David Benjamin Turitz Cox et al. Nat Med. 2015 Feb.
Abstract
Recent advances in the development of genome editing technologies based on programmable nucleases have substantially improved our ability to make precise changes in the genomes of eukaryotic cells. Genome editing is already broadening our ability to elucidate the contribution of genetics to disease by facilitating the creation of more accurate cellular and animal models of pathological processes. A particularly tantalizing application of programmable nucleases is the potential to directly correct genetic mutations in affected tissues and cells to treat diseases that are refractory to traditional therapies. Here we discuss current progress toward developing programmable nuclease-based therapies as well as future prospects and challenges.
Figures
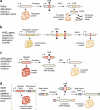
The specific type of genome editing therapy depends on the nature of the mutation causing disease. a, In gene disruption, the pathogenic function of a protein is silenced by targeting the locus with NHEJ. Formation of indels on the gene of interest often result in frameshift mutations that create premature stop codons and a non-functional protein product, or non-sense mediated decay of transcripts, suppressing gene function. c, HDR gene correction can be used to correct a deleterious mutation. A DSB is induced near the mutation site in the presence of an exogenously provided, corrective HDR template. HDR repair of the break site with the exogenous template corrects the mutation, restoring gene function. d, An alternative to gene correction is gene addition. This introduces a therapeutic transgene into either the native or a non-native locus in the genome. A DSB is induced at the desired locus and an HDR template containing homology to the break site, a promoter, a transgene and a polyadenylation (polyA) sequence is introduced to the nucleus. HDR repair recovers gene function in the target locus albeit without true physiological control over gene expression. b, In NHEJ gene correction two DSBs targeted to both sides of a pathogenic expansion or insertion may be resolved by NHEJ, causing a deletion of the intervening sequences to mediate therapy. This form of treatment would require multiplexed targeting of disease causing mutations.
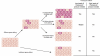
For a genome editing therapy to be efficacious, enough cells carrying the desired genome modification must exist in a tissue to reverse disease. If editing is efficient, treatment will create a population of cells carrying the desired genomic modification (depicted in pink). Depending on whether the editing event creates a fitness change in target cells, edited cells will proportionally increase, or decrease relative to unedited cells (depicted in brown) over time in tissues. Proportionally high levels of cells carrying therapeutic genome modifications in a disease-affected tissue are likely to result in a therapeutic effect. However, if low levels of a secreted gene product are needed to reverse disease, then successfully editing a small number of cells may be therapeutically efficacious.
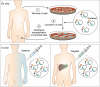
In ex vivo editing therapy cells are removed from a patient, edited and then re-engrafted (top panel). For this mode of therapy to be successful, target cells must be capable of survival outside the body and homing back to target tissues post-transplantation. In vivo therapy involves genome editing of cells in situ (bottom panels). For in vivo systemic therapy, delivery agents that are relatively agnostic to cell identity or state would be used to effect editing in a wide range of tissue types. For example systemic delivery of AAV serotype 8 vectors has been used in preclinical models to target liver tissue with high efficiency. Alternatively, in vivo therapy, may also be achieved through local injection of viral vectors to the affected tissue, such as the eye, brain, or muscle.
Similar articles
-
Recent Advances in Therapeutic Genome Editing in China.
Yang Y, Wang Q, Li Q, Men K, He Z, Deng H, Ji W, Wei Y. Yang Y, et al. Hum Gene Ther. 2018 Feb;29(2):136-145. doi: 10.1089/hum.2017.210. Hum Gene Ther. 2018. PMID: 29446996 Review.
-
Advances in targeted genome editing.
Perez-Pinera P, Ousterout DG, Gersbach CA. Perez-Pinera P, et al. Curr Opin Chem Biol. 2012 Aug;16(3-4):268-77. doi: 10.1016/j.cbpa.2012.06.007. Epub 2012 Jul 20. Curr Opin Chem Biol. 2012. PMID: 22819644 Free PMC article. Review.
-
Therapeutic genome editing with engineered nucleases.
Haas SA, Dettmer V, Cathomen T. Haas SA, et al. Hamostaseologie. 2017 Jan 31;37(1):45-52. doi: 10.5482/HAMO-16-09-0035. Epub 2017 Jan 10. Hamostaseologie. 2017. PMID: 28070592 Review.
-
Targeted genome editing tools for disease modeling and gene therapy.
Cai M, Yang Y. Cai M, et al. Curr Gene Ther. 2014 Feb;14(1):2-9. doi: 10.2174/156652321402140318165450. Curr Gene Ther. 2014. PMID: 24665839 Review.
-
Genome engineering in human cells.
Song M, Kim YH, Kim JS, Kim H. Song M, et al. Methods Enzymol. 2014;546:93-118. doi: 10.1016/B978-0-12-801185-0.00005-2. Methods Enzymol. 2014. PMID: 25398337 Review.
Cited by
-
Expression of CRISPR/Cas single guide RNAs using small tRNA promoters.
Mefferd AL, Kornepati AV, Bogerd HP, Kennedy EM, Cullen BR. Mefferd AL, et al. RNA. 2015 Sep;21(9):1683-9. doi: 10.1261/rna.051631.115. Epub 2015 Jul 17. RNA. 2015. PMID: 26187160 Free PMC article.
-
Kulbay M, Tuli N, Akdag A, Kahn Ali S, Qian CX. Kulbay M, et al. J Clin Med. 2024 Jul 19;13(14):4224. doi: 10.3390/jcm13144224. J Clin Med. 2024. PMID: 39064263 Free PMC article. Review.
-
Gene Therapy Intervention in Neovascular Eye Disease: A Recent Update.
Lin FL, Wang PY, Chuang YF, Wang JH, Wong VHY, Bui BV, Liu GS. Lin FL, et al. Mol Ther. 2020 Oct 7;28(10):2120-2138. doi: 10.1016/j.ymthe.2020.06.029. Epub 2020 Jun 30. Mol Ther. 2020. PMID: 32649860 Free PMC article. Review.
-
Suh S, Choi EH, Leinonen H, Foik AT, Newby GA, Yeh WH, Dong Z, Kiser PD, Lyon DC, Liu DR, Palczewski K. Suh S, et al. Nat Biomed Eng. 2021 Feb;5(2):169-178. doi: 10.1038/s41551-020-00632-6. Epub 2020 Oct 19. Nat Biomed Eng. 2021. PMID: 33077938 Free PMC article.
-
Mikuni T, Nishiyama J, Sun Y, Kamasawa N, Yasuda R. Mikuni T, et al. Cell. 2016 Jun 16;165(7):1803-1817. doi: 10.1016/j.cell.2016.04.044. Epub 2016 May 12. Cell. 2016. PMID: 27180908 Free PMC article.
References
-
- Lander ES. Initial impact of the sequencing of the human genome. Nature. 2011;470:187–197. - PubMed
-
- Thoene JG. Small molecule therapy for genetic disease. Cambridge University Press; Cambridge, UK ; New York: 2010.
-
- Kay MA. State-of-the-art gene-based therapies: the road ahead. Nature reviews. Genetics. 2011;12:316–328. - PubMed
-
- Gaspar HB, et al. Long-term persistence of a polyclonal T cell repertoire after gene therapy for X-linked severe combined immunodeficiency. Science translational medicine. 2011;3:97ra79. - PubMed
Publication types
MeSH terms
Substances
LinkOut - more resources
Full Text Sources
Other Literature Sources
Medical