Simultaneous recording of fluorescence and electrical signals by photometric patch electrode in deep brain regions in vivo - PubMed
- ️Thu Jan 01 2015
Simultaneous recording of fluorescence and electrical signals by photometric patch electrode in deep brain regions in vivo
Yasuharu Hirai et al. J Neurophysiol. 2015.
Abstract
Despite its widespread use, high-resolution imaging with multiphoton microscopy to record neuronal signals in vivo is limited to the surface of brain tissue because of limited light penetration. Moreover, most imaging studies do not simultaneously record electrical neural activity, which is, however, crucial to understanding brain function. Accordingly, we developed a photometric patch electrode (PME) to overcome the depth limitation of optical measurements and also enable the simultaneous recording of neural electrical responses in deep brain regions. The PME recoding system uses a patch electrode to excite a fluorescent dye and to measure the fluorescence signal as a light guide, to record electrical signal, and to apply chemicals to the recorded cells locally. The optical signal was analyzed by either a spectrometer of high light sensitivity or a photomultiplier tube depending on the kinetics of the responses. We used the PME in Oregon Green BAPTA-1 AM-loaded avian auditory nuclei in vivo to monitor calcium signals and electrical responses. We demonstrated distinct response patterns in three different nuclei of the ascending auditory pathway. On acoustic stimulation, a robust calcium fluorescence response occurred in auditory cortex (field L) neurons that outlasted the electrical response. In the auditory midbrain (inferior colliculus), both responses were transient. In the brain-stem cochlear nucleus magnocellularis, calcium response seemed to be effectively suppressed by the activity of metabotropic glutamate receptors. In conclusion, the PME provides a powerful tool to study brain function in vivo at a tissue depth inaccessible to conventional imaging devices.
Keywords: acoustic local responses; brain activity in vivo; fluorescence calcium signal; local field electrical responses; photometric patch electrode.
Copyright © 2015 the American Physiological Society.
Figures
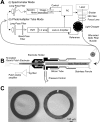
Outline of the photometric patch electrode (PME) recording system. A: laser light is transmitted through a bifurcated optic fiber bundle to the PME. The fluorescence signal is collected and analyzed by a spectrometer coupled with a charge-coupled device (CCD) camera (i) or a photomultiplier tube (PMT) coupled with a lock-in amplifier (ii). A rotating light-chopper generated a reference signal to the lock-in amplifier. PC, a personal computer to control and acquire data; I–V amp, current-voltage converter amplifier; ND Filter, neutral density filter of 25–50% transmission. B: the PME and optic fiber bundle are aligned in the holder. The holder was made airtight using a gasket (g) and a silicon tube that wrapped tightly around the electrode holder and the optic fiber bundle to control the pressure within the PME. The exterior of PME was nickel (Ni)-coated toward the proximal end. The distal end from the sharp taper to the tip was not coated (see Figs. 2A and 8A). A Teflon-coated Ag wire was inserted into the electrode through the gasket and connected to the headstage of a patch-clamp amplifier. C: the proximal end of the PME was polished. Left: polished end of the PME. Right: unpolished end of a quartz capillary.
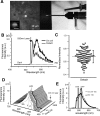
Recording of fluorescence signals in brain slices using a spectrometer. A: the PME assembly (right) and a laser-excited granule cell from the rat hippocampus (left). The inset shows the laser-illuminated neuron at a larger magnification with a calibration bar of 10 μm where the PME tip is indicated by dotted lines. B: 2 fluorescence spectra recorded from a dye-filled nucleus magnocellularis (NM) neuron of the chick by the on-cell and detached PME. Cells were labeled with Alexa Fluor 555 dextran (10-kDa mol mass) and were excited by a 532-nm laser. a.u., Arbitrary units. C: the percentage of the drop in fluorescence intensity after detachment of the PME was plotted for individual cells. After detachment of the PME, the fluorescence intensity dropped to approximately 42–88% (68 ± 9.5%, n = 115 cells). The difference signal was not affected by the type of fluorophore; thus data from various dyes were combined. D: a stacked display of time series spectra shows fluorescence resonance energy transfer (FRET) responses from a rat cortical pyramidal neuron after subtraction of the dark level (Dark in B). The spectrum was sampled at 20-s intervals, and every other data are plotted. Spectra before (dotted line) and after (thick black solid line) the bath application of a high-KCl medium (30 mM; arrow) are indicated in D and E. The background spectrum (BG; gray broken line) was overlaid on the 1st time series record. Arrows Y and C, peaks of enhanced yellow fluorescent protein (eYFP) and enhanced cyan fluorescent protein (eCFP), respectively. E: the BG was subtracted to show the spectrum change induced by KCl. Vertical gray bars indicate the wavelength ranges used to calculate eCFP and eYFP fluorescence intensity. In A and D, neurons were transfected by an eCFP-eYFP-based FRET compound and were excited by a 445-nm laser (Takatsuka et al. 2005).
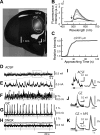
Oregon Green BAPTA-1 (OGB-1) AM spectral signals from the auditory cortex (field L). A: a frontal section of chick cerebral cortex containing field L. The fluorescence image is superimposed on a bright-field image. MPall, medial pallium; LPall, lateral palllium; D, dorsal; L, lateral. The vertical broken line indicates the midline. B: the spectrum increased when the PME approached the field L (black line on the brain surface and thick gray line at the field L). The field L was preloaded with OGB-1 AM. The broken line indicates the base level (Fdark). The arrow indicates the 488-nm laser peak. C: an increase of fluorescence in B. The electrode approaching was stopped when the fluorescence intensity became stable. D and E: the spontaneous local field current recorded with artificial cerebrospinal fluid (ACSF)-filled PME (D) with the boxed area expanded (E). F: local field current (black) and overlaid fluorescence (gray). Binaural acoustic stimulation (80-dB white noise; gray line at the bottom) was applied unless otherwise specified. G: gabazine (GZ; 5 mM) enhanced responses. H: 6,7-dinitroquinoxaline-2,3-dione (DNQX; 50 mM) blocked field responses. I and J: current (black) and fluorescence (gray) signals correspond to asterisks in F (I) and G (J). The local field current was induced in conjunction with the acoustic stimulus and is overlaid (gray with dots) in J and K. K: the current (black) and fluorescence (gray) signals recorded in 2-amino-5-phosphonopentanoic acid (AP5; 25 mM) and gabazine (5 mM).
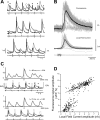
Correlation between current and fluorescence signals in the field L. Fluorescence was detected by a photomultiplier tube in gabazine (0.5 mM). A: local field currents (black) and fluorescence responses (gray) to acoustic stimuli (gray line at the bottom). Current and fluorescence responses were generally coupled. Uncoupled responses are indicated by ○ or ▼ (see main text). B: the ensemble averages of fluorescence (top) and current (bottom) signals. Individual traces are overlaid. C: 2 examples of the cross-correlogram between current and fluorescence signals. From top to bottom, each subpanel illustrates the cross-correlogram, fluorescence, current traces, and acoustic stimulus. In the correlogram, t indicates the delay time, and x.c. represents the correlation coefficient between 2 traces. The short black line at the right end of the fluorescence signal indicates the time displaced (delay time) to align the current and fluorescence signals to measure the peaks of a pair (boxes). D: scatter plots of paired fluorescence (F) and current amplitudes. Both acoustic and spontaneous responses are included. Liner fit slope is 0.94%/nA (r = 0.58, lower group) and 0.60%/nA (r = 0.76, upper group).
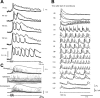
Acoustic responses of the field L recorded by a photomultiplier tube with 4-aminopyridine (4-AP). A: example traces of local field current (black) and fluorescence (gray). Time indicated is after the start of the local application of 4-AP (5 mM) through the PME. B: 15 min after 4-AP application burst responses occurred. The number on each trace indicates the time after the start of the recording session. Individual traces were sampled at 7-s intervals. A single burst continued for 77 s with approximately 126- to 133-s intervals. A total of 4 bursts occurred during the 777-s recording time. C: local field current and fluorescence signals responded strongly to contralateral or binaural sound stimuli. Individual local current traces and ensemble averages are overlaid. Fluorescence signals are illustrated after ensemble-averaging.
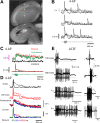
Local responses in the inferior colliculus (IC). A: a frontal section to show the recording site at a depth of 7 mm in the IC. Arrowheads indicate the electrode track of the OGB-1 AM labeling. *Injection site. B–D: the acoustic response was recorded using a photomultiplier tube with 20 mM 4-AP in the PME. B: the acoustic response followed by spontaneous activity. C: ensemble-averaged responses to binaural (dotted red), contralateral (green), or ipsilateral (black) stimulation. Local field currents are overlaid for 3 conditions. D: fluorescence (dotted line) and current (solid line) responses are overlaid in the top 2 traces. Stimulus duration is indicated by colors. In the bottom 2 traces, ensemble-averaged fluorescence and current signals are overlaid separately. E: single-trial local currents of single-unit activity (black) and fluorescence response (gray; right) recorded with an ACSF-filled PME using a spectrometer. The stimulus duration was changed (overlaid gray; left). Fluorescence responses were recorded with a sampling rate of 0.5 s (top) and 0.3 s (3 other traces) and were overlaid with the local current response (black) of the pair.
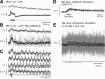
Nucleus magnocellularis (NM) responses to acoustic stimulation in vivo or to electrical stimulation in slices as recorded by a photomultiplier tube. A: ensemble-averaged acoustic responses of local field current (black) and OGB-1 AM fluorescence (gray) recorded in vivo with a 4-AP-filled PME (5 mM). B and C: acoustic responses recorded with 4-AP (10 mM), TEA (10 mM), and gabazine (0.5 mM) in B (ensemble-averaged) and C (single traces from the data set of B). D: the fluorescence signal induced by antidromic electrical stimulation of NM projection fibers to the nucleus laminaris was recorded in a brain-stem slice (300 μm) bathed either in ACSF (black) or in ACSF with 0.25 mM 4-AP (gray). E: the fluorescence signal was recorded in response to orthodromic electrical stimulation to auditory nerve fibers in a brain-stem slice bathed in ACSF with LY341495 (1 μM) and 4-AP (0.25 mM; dotted line, overlaid with individual traces in gray) or in 4-AP (0.25 mM in ACSF; solid line). Electrical stimulation (a train of 20 stimuli, 100 μs at 100 Hz) was applied in D and E, and signals were ensemble-averaged. OGB-1 AM was loaded by in vivo injection, and recordings were made using a cell-attached PME filled with ACSF in D and E.
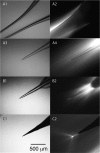
Laser leaks imaged under 3 different PME surface coatings. A pair of images was obtained in a bathing solution containing Alexa Fluor 488 either under bright-field illumination (left) or 488-nm laser illumination through the PME (right). A: the PME was coated with Ni toward the thick proximal end; however, the PME had a bare surface from the sharp taper to the tip (A1 and A3). The laser leak occurred at the sharp taper (A2). The tip of PME is indicated by a dotted line (A4) because the tip is invisible due to the strong background fluorescence. B: a Sylgard coating was applied close to the tip of PME (B1). The laser leak emerged from the edge of the Sylgard coat, but the background fluorescence was reduced, and the emission of laser light from the tip was visible as a fluorescence spot (B2). C: the PME was coated close to the tip with Sylgard mixed with soot (C1). The background fluorescence was reduced, and limited laser emission occurred at the distal taper near the tip and at the tip end of the electrode (C2).
Similar articles
-
Ohmori H, Hirai Y, Matsui R, Watanabe D. Ohmori H, et al. J Neurosci Methods. 2023 May 15;392:109863. doi: 10.1016/j.jneumeth.2023.109863. Epub 2023 Apr 17. J Neurosci Methods. 2023. PMID: 37075913
-
Optical detection of neuron connectivity by random access two-photon microscopy.
Shafeghat N, Heidarinejad M, Murata N, Nakamura H, Inoue T. Shafeghat N, et al. J Neurosci Methods. 2016 Apr 1;263:48-56. doi: 10.1016/j.jneumeth.2016.01.023. Epub 2016 Feb 3. J Neurosci Methods. 2016. PMID: 26851307
-
Du C, Pan Y. Du C, et al. Rev Neurosci. 2011;22(6):695-709. doi: 10.1515/RNS.2011.053. Epub 2011 Nov 18. Rev Neurosci. 2011. PMID: 22098474 Review.
-
Optical monitoring of brain function in vivo: from neurons to networks.
Garaschuk O, Milos RI, Grienberger C, Marandi N, Adelsberger H, Konnerth A. Garaschuk O, et al. Pflugers Arch. 2006 Dec;453(3):385-96. doi: 10.1007/s00424-006-0150-x. Epub 2006 Oct 18. Pflugers Arch. 2006. PMID: 17047983 Review.
Cited by
-
Optogenetics Identification of a Neuronal Type with a Glass Optrode in Awake Mice.
Ono M, Muramoto S, Ma L, Kato N. Ono M, et al. J Vis Exp. 2018 Jun 28;(136):57781. doi: 10.3791/57781. J Vis Exp. 2018. PMID: 30010633 Free PMC article.
-
Automated microscope-independent fluorescence-guided micropipette.
Miranda C, Howell MR, Lusk JF, Marschall E, Eshima J, Anderson T, Smith BS. Miranda C, et al. Biomed Opt Express. 2021 Jul 6;12(8):4689-4699. doi: 10.1364/BOE.431372. eCollection 2021 Aug 1. Biomed Opt Express. 2021. PMID: 34513218 Free PMC article.
References
-
- Akerboom J, Chen TW, Wardill TJ, Tian L, Marvin JS, Mutlu S, Calderón NC, Esposti F, Borghuis BG, Sun XR, Gordus A, Orger MB, Portugues R, Engert F, Macklin JJ, Filosa A, Aggarwal A, Kerr RA, Takagi R, Kracun S, Shigetomi E, Khakh BS, Baier H, Lagnado L, Wang SS, Bargmann CI, Kimmel BE, Jayaraman V, Svoboda K, Kim DS, Schreiter ER, Looger LL. Optimization of a GCaMP calcium indicator for neural activity imaging. J Neurosci 32: 13819–13840, 2012. - PMC - PubMed
-
- Berridge MJ, Lipp P, Bootman MD. The versatility and universality of calcium signaling. Nat Rev Mol Cell Biol 1: 11–21, 2000. - PubMed
-
- Biederman-Thorson M. Auditory evoked responses in the cerebrum (Field-L) and ovoid nucleus of the ring dove. Brain Res 24: 235–245, 1970. - PubMed
Publication types
MeSH terms
Substances
LinkOut - more resources
Full Text Sources
Other Literature Sources
Research Materials