Chitin is endogenously produced in vertebrates - PubMed
- ️Thu Jan 01 2015
Chitin is endogenously produced in vertebrates
W Joyce Tang et al. Curr Biol. 2015.
Abstract
Chitin, a biopolymer of N-acetylglucosamine, is abundant in invertebrates and fungi and is an important structural molecule [1, 2]. There has been a longstanding belief that vertebrates do not produce chitin; however, we have obtained compelling evidence to the contrary. Chitin synthase genes are present in numerous fishes and amphibians, and chitin is localized in situ to the lumen of the developing zebrafish gut, in epithelial cells of fish scales, and in at least three different cell types in larval salamander appendages. Chitin synthase gene knockdowns and various histochemical experiments in zebrafish further authenticated our results. Finally, a polysaccharide was extracted from scales of salmon that exhibited all the chemical hallmarks of chitin. Our data and analyses demonstrate the existence of endogenous chitin in vertebrates and suggest that it serves multiple roles in vertebrate biology.
Copyright © 2015 Elsevier Ltd. All rights reserved.
Figures
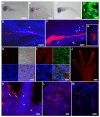
Detection of chitin in zebrafish and axolotl. (A-D) in situ hybridization for DrCHS-1. NBT/BCIP signals were observed primarily in the developing digestive tract in 5 dpf (A) and 10 dpf (B) larvae, indicating that DrCHS-1 is expressed during larval development and in the gut. A representative “sense” control larva is shown in (C). No NBT/BCIP signals were observed in the developing gut of control larvae at either 5 or 10 dpf, although background staining was observed in the cranial region. Scale bars = 500 μm. (D) The ventral region of the mid-intestine of a 10 dpf larva (transverse section, different specimen than (B)), shows DrCHS-1 expression in select cells within the columnar intestinal epithelium (arrowheads) and in mesenchymal cells adjacent to the intestinal epithelium (arrow). NBT/BCIP deposition was imaged in the infrared range with confocal microscopy (blue), and nuclei were stained with Sytox Green (green). Scale bar = 10 μm. (E-M) A chitin probe (CBD-546, shown in red) was used for affinity histochemistry on fixed whole mount specimens of zebrafish larvae (E-G) and scales (H-I), and axolotl larval forelimbs and tails (J-M). Nuclei were stained with TOTO-3 (blue), and rostral is to the left in (E) and (F). (E) 3D-rendered projection of a 4 dpf zebrafish larva. “f” and “m” represent preanal finfold and somitic muscle, respectively; the “m” designation is above the approximate junction of the middle intestine. Chitin is observed in the developing larval gut, appearing fibrillar in the lumen of the intestinal bulb and coalescing as the lumen narrows posteriorly. Scale bar = 100 μm. (F) 3D-rendered projection of the mid-intestinal lumen from a 5 dpf zebrafish larva. The fibrous nature of the chitin is obvious and several cells within the intestinal epithelial wall are observed that show strong chitin signals (arrowheads). A population of mesenchymal cells was also found to be positive for chitin signals (arrow). Scale bar = 20 μm. (G) Transverse section from a 3D volume-rendered reconstruction of the image in (F) showing chitin distributed throughout the lumen of the mid-intestine. (H) Confocal image of a field of zebrafish scale epithelium. Panels shown are: CBD-546 (top left), DIC (top right), Sytox Green nuclear counterstain (pseudocolored in blue, bottom left), and merged image (bottom right). The four circular structures with red chitin signals are goblet cells that show polarized, crescent-shaped nuclei; polygonal concentric structures on the DIC overlay are squamous epithelial cells. Aggregations of small red punctate spots probably represent extracellular chitin. (I) Maximum projection image of a field of scale epithelial cells in which chitin is prominent throughout the cytoplasm of club cells. Panels shown are: CBD-546 (top left), phalloidin-FITC (top right), TOTO-3 nuclear counterstain (bottom left), and merged image (bottom right). The scale specimen was simultaneously stained for F-actin using phalloidin-FITC to help distinguish individual cells. Scale bars = 10 μm (H, I). (J) A forelimb (palm side up) of a larval axolotl after chitin histochemistry, imaged on a stereoscope. The tips of the developing digits and the palm area that show extensive chitin signals are primarily superficial epithelial cells. Scale bar = 200 μm. (K) A field from the base of a digit from a different forelimb than shown in (J) imaged by confocal microscopy. Arrowheads indicate several specialized Leydig epidermal cells, and the arrows identify a few of the superficial epithelial cells seen in (J). (L,M) Caudal fin of larval axolotl. (L) Maximum intensity projection showing a subset of surface epithelial cells that exhibit strong chitin signals. (M) A deeper optical section showing strong chitin signals in the fibroblast-like cells of the fin mesenchyme. Scale bars = 50 μm (K,L,M). See also Figures S1, S2.
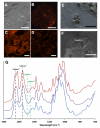
Extraction and analysis of chitin from scale material of Atlantic salmon. (A-D) Skin (including scales) from a specimen of farmed Atlantic salmon was subjected to chitin extraction following procedures outlined in Methods (Supplemental Experimental Procedures). After extraction, the precipitated pellets were collected, stained for chitin with the CBD-546 probe and imaged using an epifluorescent stereomicroscope. Scale bars = 500 μm. (A) A representative pellet from the chitin extraction, photographed using visible light only. (B) CBD-546 chitin detection of the same pellet as in (A), showing strong punctate signals amongst a background of unstained or weakly stained material which has been shown to be comprised largely of deacetylated chitin. Controls included purified α-chitin from shrimp shells (C) and chitosan (75-85% deacetylated shrimp shell chitin) (D). The controls demonstrate that our CBD-546 probe is very specific to intact chitin as it did not give appreciable signals with chitosan. (E) Stereomicrograph of an area containing several chitinous granules within the thin, acid-soluble film. Scale bar = 100 μm. (F) SEM image of a chitinous granule. (G) FTIR analysis of the fingerprint region of the chitinous granules (blue) and its comparison with β-chitin from squid pen (purple) and α-chitin from shrimp shells (red). Two additional resonances -- 1494 cm−1 and 1453 cm−1 -- stand out as main differences of our extracted chitin with respect to the controls and are diagnostic of aromatic ring breathing modes that were subsequently identified as polystyrene contamination of the salmon material (discussed in Supplemental Information). Scale bar = 40 μm.
Similar articles
-
Evidence of chitin in the ampullae of Lorenzini of chondrichthyan fishes.
Phillips M, Tang WJ, Robinson M, Daza DO, Hassan K, Leppert V, Hirst LS, Amemiya CT. Phillips M, et al. Curr Biol. 2020 Oct 19;30(20):R1254-R1255. doi: 10.1016/j.cub.2020.08.014. Curr Biol. 2020. PMID: 33080193
-
Hogenkamp DG, Arakane Y, Zimoch L, Merzendorfer H, Kramer KJ, Beeman RW, Kanost MR, Specht CA, Muthukrishnan S. Hogenkamp DG, et al. Insect Biochem Mol Biol. 2005 Jun;35(6):529-40. doi: 10.1016/j.ibmb.2005.01.016. Epub 2005 Mar 7. Insect Biochem Mol Biol. 2005. PMID: 15857759
-
Evolution and multi-functionality of the chitin system.
Wagner GP. Wagner GP. EXS. 1994;69:559-77. doi: 10.1007/978-3-0348-7527-1_33. EXS. 1994. PMID: 7994124 Review.
-
Zhang Y, Foster JM, Nelson LS, Ma D, Carlow CK. Zhang Y, et al. Dev Biol. 2005 Sep 15;285(2):330-9. doi: 10.1016/j.ydbio.2005.06.037. Dev Biol. 2005. PMID: 16098962
-
Function of chitin oligosaccharides in plant and animal development.
Bakkers J, Kijne JW, Spaink HP. Bakkers J, et al. EXS. 1999;87:71-83. doi: 10.1007/978-3-0348-8757-1_5. EXS. 1999. PMID: 10906952 Review.
Cited by
-
Harðardóttir HM, Male R, Nilsen F, Dalvin S. Harðardóttir HM, et al. Life (Basel). 2021 Jan 13;11(1):47. doi: 10.3390/life11010047. Life (Basel). 2021. PMID: 33450932 Free PMC article.
-
Liberti A, Natarajan O, Atkinson CGF, Sordino P, Dishaw LJ. Liberti A, et al. Front Immunol. 2021 Feb 25;12:642687. doi: 10.3389/fimmu.2021.642687. eCollection 2021. Front Immunol. 2021. PMID: 33717199 Free PMC article. Review.
-
Aqueous Modification of Chitosan with Itaconic Acid to Produce Strong Oxygen Barrier Film.
Sirviö JA, Kantola AM, Komulainen S, Filonenko S. Sirviö JA, et al. Biomacromolecules. 2021 May 10;22(5):2119-2128. doi: 10.1021/acs.biomac.1c00216. Epub 2021 Apr 29. Biomacromolecules. 2021. PMID: 33913322 Free PMC article.
-
Skåne A, Edvardsen PK, Cordara G, Loose JSM, Leitl KD, Krengel U, Sørum H, Askarian F, Vaaje-Kolstad G. Skåne A, et al. BMC Microbiol. 2022 Aug 8;22(1):194. doi: 10.1186/s12866-022-02590-2. BMC Microbiol. 2022. PMID: 35941540 Free PMC article.
-
Zhang M, Chen H, Liu L, Xu L, Wang X, Chang L, Chang Q, Lu G, Jiang J, Zhu L. Zhang M, et al. Front Microbiol. 2020 Mar 3;11:162. doi: 10.3389/fmicb.2020.00162. eCollection 2020. Front Microbiol. 2020. PMID: 32194513 Free PMC article.
References
-
- Ng AN, de Jong-Curtain TA, Mawdsley DJ, White SJ, Shin J, Appel B, Dong PD, Stainier DY, Heath JK. Formation of the digestive system in zebrafish: III. Intestinal epithelium morphogenesis. Dev Biol. 2005;286:114–135. - PubMed
-
- Muzzarelli RAA, Boudrant J, Meyer D, Manno N, DeMarchis M, Paoletti MG. Current views on fungal chitin/chitosan, human chitinases, food preservation, glucans, pectins and inulin: A tribute to Henri Braconnot, precursor of the carbohydrate polymers science, on the chitin bicentennial. Carbohydrate Polymers. 2012;87:995–1012.
Publication types
MeSH terms
Substances
LinkOut - more resources
Full Text Sources
Other Literature Sources
Molecular Biology Databases