Rotenone Stereospecifically Increases (S)-2-Hydroxyglutarate in SH-SY5Y Neuronal Cells - PubMed
- ️Thu Jan 01 2015
. 2015 May 18;28(5):948-54.
doi: 10.1021/tx500535c. Epub 2015 Apr 8.
Affiliations
- PMID: 25800467
- PMCID: PMC4721232
- DOI: 10.1021/tx500535c
Rotenone Stereospecifically Increases (S)-2-Hydroxyglutarate in SH-SY5Y Neuronal Cells
Andrew J Worth et al. Chem Res Toxicol. 2015.
Abstract
The α-ketoglutarate metabolite, 2-hydroxyglutarate (2-HG), has emerged as an important mediator in a subset of cancers and rare inherited inborn errors of metabolism. Because of potential enantiospecific metabolism, chiral analysis is essential for determining the biochemical impacts of altered 2-HG metabolism. We have developed a novel application of chiral liquid chromatography-electron capture/atmospheric pressure chemical ionization/mass spectrometry, which allows for the quantification of both (R)-2-HG (D-2-HG) and (S)-2-HG (L-2-HG) in human cell lines. This method avoids the need for chiral derivatization, which could potentially distort enantiomer ratios through racemization during the derivatization process. The study revealed that the pesticide rotenone (100 nM), a mitochondrial complex I inhibitor, caused a significant almost 3-fold increase in the levels of (S)-2-HG, (91.7 ± 7.5 ng/10(6) cells) when compared with the levels of (R)-2-HG (24.1 ± 1.2 ng/10(6) cells) in the SH-SY5Y neuronal cells, a widely used model of human neurons. Stable isotope tracers and isotopologue analysis revealed that the increased (S)-2-HG was derived primarily from l-glutamine. Accumulation of highly toxic (S)-2-HG occurs in the brains of subjects with reduced L-2-HG dehydrogenase activity that results from mutations in the L2HGDH gene. This suggests that the observed stereospecific increase of (S)-2-HG in neuronal cells is due to rotenone-mediated inhibition of L-2-HG dehydrogenase but not D-2-HG dehydrogenase. The high sensitivity chiral analytical methodology that has been developed in the present study can also be employed for analyzing other disruptions to 2-HG formation and metabolism such as those resulting from mutations in the isocitrate dehydrogenase gene.
Conflict of interest statement
Notes
The authors declare no competing financial interest.
Figures
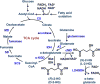
Schematic of the TCA cycle. Abbreviations: α-KGD, α-ketoglutarate dehydrogenase; AT, aspartate transaminase; CS, citrate synthase; L2HGDH, L-2-hydroxyglutarate dehydrogenase; MD, malate dehydrogenase; MutIDH, mutated isocitrate dehydrogenase; SCS, succinyl-CoA synthase; SD, succinate dehydrogenase.
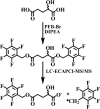
Formation of 2-HG-bis-PFB followed by LC-ECAPCI/MS/MS.
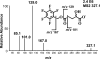
Product ions derived from LC-ECAPCI/MS/MS analysis of [M-PFB]− at m/z 327 derived from 2-HG-bis-PFB.
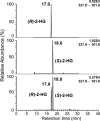
Chromatographic separation of (R)-2-HG from (S)-2-HG using normal-phase chiral LC-ECAPCI/MS.
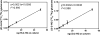
Calibration curves for (R)-2-HG and (S)-2-HG. (R)-2-HG: y = 0.0021x + 0.0056, r2 = 0.995. (S)-2-HG: y = 0.0022x + 0.0046, r2 = 0.988.
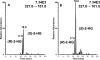
Separation of (R)-2-HG and (S)-2-HG from SH-SY5Y neuronal cells using normal-phase chiral LC-ECAPCI/MS. The cells were treated with (A) DMSO and (B) 100 nM rotenone.
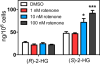
Rotenone induces stereospecific increases in (S)-2-HG. Student’s two-tailed t test against vehicle control: *p < 0.05, **p < 0.001 (n = 4). Rotenone induced a stereoselective increase in (S)-2-HG when compared with (R)-2-HG. SH-SY5Y cells were treated with 100 nM rotenone or vehicle (DMSO) for 6 h. Student’s two-tailed t test for (S)-2-HG against (R)-2-HG after rotenone treatment: **p = 0.0016 (n = 4).
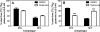
Glutamine contributes to increased (S)-2-HG production in response to rotenone. SH-SY5Y cells were grown in the presence of 2 mM L-[13C515N2]-glutamine and either 100 nM rotenone or vehicle DMSO for 6 h followed by isotopic enrichment analysis by LC-ECAPCI-MS/MS. Student’s two-tailed t test against vehicle control: ***p < 0.001 (n = 4).
Similar articles
-
L-2-hydroxyglutaric aciduria, a defect of metabolite repair.
Rzem R, Vincent MF, Van Schaftingen E, Veiga-da-Cunha M. Rzem R, et al. J Inherit Metab Dis. 2007 Oct;30(5):681-9. doi: 10.1007/s10545-007-0487-0. Epub 2007 Jun 21. J Inherit Metab Dis. 2007. PMID: 17603759
-
Zheng JY, Jiang X, Zhou JL, Shi ZQ, Liu LF, Xin GZ. Zheng JY, et al. Anal Chim Acta. 2019 Oct 24;1077:174-182. doi: 10.1016/j.aca.2019.05.056. Epub 2019 May 25. Anal Chim Acta. 2019. PMID: 31307707
-
2-Hydoxyglutarate: D/Riving Pathology in gLiomaS.
Wahl DR, Venneti S. Wahl DR, et al. Brain Pathol. 2015 Nov;25(6):760-8. doi: 10.1111/bpa.12309. Brain Pathol. 2015. PMID: 26526944 Free PMC article. Review.
-
D-2-Hydroxyglutarate in Glioma Biology.
Chou FJ, Liu Y, Lang F, Yang C. Chou FJ, et al. Cells. 2021 Sep 7;10(9):2345. doi: 10.3390/cells10092345. Cells. 2021. PMID: 34571995 Free PMC article. Review.
Cited by
-
Chen F, Bian K, Tang Q, Fedeles BI, Singh V, Humulock ZT, Essigmann JM, Li D. Chen F, et al. Chem Res Toxicol. 2017 Apr 17;30(4):1102-1110. doi: 10.1021/acs.chemrestox.7b00009. Epub 2017 Mar 24. Chem Res Toxicol. 2017. PMID: 28269980 Free PMC article.
-
The α-ketoglutarate dehydrogenase complex in cancer metabolic plasticity.
Vatrinet R, Leone G, De Luise M, Girolimetti G, Vidone M, Gasparre G, Porcelli AM. Vatrinet R, et al. Cancer Metab. 2017 Feb 2;5:3. doi: 10.1186/s40170-017-0165-0. eCollection 2017. Cancer Metab. 2017. PMID: 28184304 Free PMC article. Review.
-
Oncometabolites and the response to radiotherapy.
Xiang K, Jendrossek V, Matschke J. Xiang K, et al. Radiat Oncol. 2020 Aug 14;15(1):197. doi: 10.1186/s13014-020-01638-9. Radiat Oncol. 2020. PMID: 32799884 Free PMC article. Review.
-
Oldham WM, Clish CB, Yang Y, Loscalzo J. Oldham WM, et al. Cell Metab. 2015 Aug 4;22(2):291-303. doi: 10.1016/j.cmet.2015.06.021. Epub 2015 Jul 23. Cell Metab. 2015. PMID: 26212716 Free PMC article.
-
Gondáš E, Baranovičová E, Bystrický P, Šofranko J, Evinová A, Dohál M, Hatoková Z, Murín R. Gondáš E, et al. Neurochem Res. 2024 Sep;49(9):2480-2490. doi: 10.1007/s11064-024-04188-8. Epub 2024 Jun 12. Neurochem Res. 2024. PMID: 38862727 Free PMC article.
References
Publication types
MeSH terms
Substances
Grants and funding
LinkOut - more resources
Full Text Sources
Other Literature Sources