Eag Domains Regulate LQT Mutant hERG Channels in Human Induced Pluripotent Stem Cell-Derived Cardiomyocytes - PubMed
- ️Thu Jan 01 2015
Eag Domains Regulate LQT Mutant hERG Channels in Human Induced Pluripotent Stem Cell-Derived Cardiomyocytes
Qiang-Ni Liu et al. PLoS One. 2015.
Abstract
Human Ether á go-go Related Gene potassium channels form the rapid component of the delayed-rectifier (IKr) current in the heart. The N-terminal 'eag' domain, which is composed of a Per-Arnt-Sim (PAS) domain and a short PAS-cap region, is a critical regulator of hERG channel function. In previous studies, we showed that isolated eag (i-eag) domains rescued the dysfunction of long QT type-2 associated mutant hERG R56Q channels, by substituting for defective eag domains, when the channels were expressed in Xenopus oocytes or HEK 293 cells.Here, our goal was to determine whether the rescue of hERG R56Q channels by i-eag domains could be translated into the environment of cardiac myocytes. We expressed hERG R56Q channels in human induced pluripotent stem cell-derived cardiomyocytes (hiPSC-CMs) and measured electrical properties of the cells with whole-cell patch-clamp recordings. We found that, like in non-myocyte cells, hERG R56Q had defective, fast closing (deactivation) kinetics when expressed in hiPSC-CMs. We report here that i-eag domains slowed the deactivation kinetics of hERG R56Q channels in hiPSC-CMs. hERG R56Q channels prolonged the AP of hiPSCs, and the AP was shortened by co-expression of i-eag domains and hERG R56Q channels. We measured robust Förster Resonance Energy Transfer (FRET) between i-eag domains tagged with Cyan fluorescent protein (CFP) and hERG R56Q channels tagged with Citrine fluorescent proteins (Citrine), indicating their close proximity at the cell membrane in live iPSC-CMs. Together, functional regulation and FRET spectroscopy measurements indicated that i-eag domains interacted directly with hERG R56Q channels in hiPSC-CMs. These results mean that the regulatory role of i-eag domains is conserved in the cellular environment of human cardiomyocytes, indicating that i-eag domains may be useful as a biological therapeutic.
Conflict of interest statement
Competing Interests: The authors have declared that no competing interests exist.
Figures
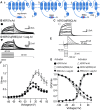
A, Schematic depicting hERG channel subunit: a, WT hERG1a; b, hERG1a(R56Q); c, hERG1a(R56Q) + i-eag. B, C, D, Representative current recordings from HEK293 cells expressing: WT hERG1a.Ad, hERG1a(R56Q).Ad, hERG1a(R56Q).Ad + i-eag.Ad. Inset represents the voltage protocol used. E, IV relationships of WT hERG1a, hERG1a(R56Q), and hERG1a(R56Q).Ad + i-eag.Ad. The current at the end of the depolarizing step was normalized to the maximum tail current and plotted versus voltage. F, Representative steady-state inactivation current recording from WT hERG1a (top) using a three-pulse protocol (bottom). G, Steady-state activation and inactivation curves. Steady-state activation curves were generated by normalizing tail currents at -50 mV to the maximum tail current and plotted versus voltage. Steady-state inactivation curves were generated by normalizing the peak current and plotted versus voltage. Both steady-state activation and inactivation curves were fit with a Boltzmann function. All values were plotted as mean ± S.E.M; n = 5–13 cells.
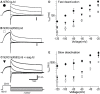
Representative tail current recordings from HEK293 cells expressing: A, WT hERG1a.Ad; B, hERG1a(R56Q).Ad; C, hERG1a(R56Q).Ad + i-eag.Ad. Inset represents the voltage protocol used. D, Tail currents were fit with a double exponential function and the mean τfast (top) and τslow (bottom) values were plotted against voltage on a logarithmic scale. All values were plotted as mean ± S.E.M; n = 6–8 cells.
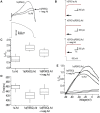
Representative current recordings: A (top) from WT hERG1a (black), hERG1a R56Q (red), and hERG R56Q + i-eag (blue) elicited with a voltage command mimicking a ventricular action potential A (bottom). B, Tail currents were generated by a step to −100 from 60 mV from same cells as in A). Double exponential fits (red traces) were extrapolated back to the moment of voltage change to obtain the peak tail current value (arrow). C, Box plot of peak currents elicited with AP command voltage (as in A) were normalized to peak tail current (as in B) to normalize for variations in channel expression between cells. D, Box plot of the time of the peak current elicited with the AP command voltage. For box plots, the middle line is the mean, the top and bottom lines are the 75th and 25th percentiles, respectively, and the straight lines are the 90th and 10th percentiles. n = 6–9 cells.E, Plot of currents in A) versus AP command voltage.
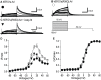
Voltage-clamp recordings of hERG currents measured from hiPSC-CMs infected by: A, WT hERG1a.Ad; B, hERG1a(R56Q).Ad; C, hERG1a(R56Q).Ad + i-eag.Ad. Inset represents the protocol used. D, I-V relationships for WT hERG1a.Ad, hERG1a(R56Q).Ad and hERG1a(R56Q).Ad + i-eag.Ad. The current at the end of depolarizing step was normalized to the maximum tail current and plotted versus voltage. All values were plotted as mean ± S.E.M; n> = 15 cells.
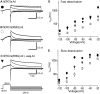
Representative tail currents recorded from hiPSC-CMs infected by: A, WT hERG1a.Ad; B, hERG1a(R56Q).Ad; C, hERG1a(R56Q).Ad + i-eag.Ad. Inset represents the voltage protocol used. D, Tails were fit with a double exponential function, and mean τfast (top) and τslow (bottom) values were plotted against voltage on a logarithmic scale. All values were plotted as mean ± S.E.M.; n> = 15 cells.
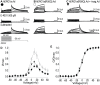
hERG currents overexpressed in hiPSC-CMs were isolated as E-4031-sensitive currents. A,B,C, The data showed families of current traces for WT hERG1a.Ad, hERG1a(R56Q).Ad and hERG1a(R56Q) + i-eag.Ad (top), after the addition of E4031(500 μM; Middle), and digitally subtracted traces to give the E-4031 sensitive currents (bottom). D, I-V relationships for each hERG channel above. The current at the end of depolarizing step was normalized to the maximum tail current and plotted versus voltage. E, Steady-state activation curves for each hERG channel above. All values were plotted as mean ± S.E.M.; n> = 15 cells.
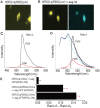
Images of cells: A, hERG1a(R56Q).Citrine.Ad; B, hERG1a(R56Q).Citrine.Ad + i-eag.CFP.Ad. C, Determination of Ratio A0. The emission spectra from hiPSCs expressing hERG1a(R56Q).Citrine was determined with excitation at 436 nm and 500 nm. Ratio A0 is the F436 spectra normalized to F500 spectra. D, Spectra method for measuring FRET and determining Ratio A. Spectra (dark blue trace) was measured from cells coexpressing i-eag.CFP.Ad + hERG1a(R56Q).Citrine.Ad by excitation at 436nm. Emission spectra of CFP (cyan) were measured in a control experiment from hiPSCs expressing i-eag.CFP. Extracted spectra (F436, red trace) is the cyan spectra subtracted from the dark blue trace and contains the emission of Citrine. Spectrum (F500, black trace) was measured from excitation of Citrine at 500 nm. Ratio A is the F436 spectra normalized to F500 spectra. E, Bar graph of Ratio A- Ratio A0, a value directly related to FRET efficiency. n = 7–9 cells. *p<0.05 for FRET between hERG1a(R56Q) and i-eag-CFP domains compared to hERG1a and i-eag-CFP control.
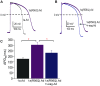
Current clamp recording of action potentials from hiPSC-CMs expressing WT hERG1a.Ad, hERG1a(R56Q).Ad and hERG1a(R56Q).Ad + i-eag.Ad. A, hERG1a(R56Q) increased the action potential duration (*p <0.05 compared to WT hERG1a.Ad). B, Coexpression of i-eag.Ad decreased the action potential duration in cells with hERG1a(R56Q) (*p <0.05 compared to hERG1a(R56Q).Ad). C, Histogram of APD90 values in hiPSC-CMs infected by WT hERG1a.Ad, hERG1a(R56Q).Ad, and hERG1a(R56Q).Ad + i-eag.Ad. n = 12 for each. Scale bar is 50 s and 20 mV.
Similar articles
-
Gustina AS, Trudeau MC. Gustina AS, et al. Proc Natl Acad Sci U S A. 2009 Aug 4;106(31):13082-7. doi: 10.1073/pnas.0900180106. Epub 2009 Jul 27. Proc Natl Acad Sci U S A. 2009. PMID: 19651618 Free PMC article.
-
Gianulis EC, Trudeau MC. Gianulis EC, et al. J Biol Chem. 2011 Jun 24;286(25):22160-9. doi: 10.1074/jbc.M110.205948. Epub 2011 May 2. J Biol Chem. 2011. PMID: 21536673 Free PMC article.
-
Gianulis EC, Liu Q, Trudeau MC. Gianulis EC, et al. J Gen Physiol. 2013 Oct;142(4):351-66. doi: 10.1085/jgp.201310995. Epub 2013 Sep 16. J Gen Physiol. 2013. PMID: 24043860 Free PMC article.
-
HERG potassium channel regulation by the N-terminal eag domain.
Gustina AS, Trudeau MC. Gustina AS, et al. Cell Signal. 2012 Aug;24(8):1592-8. doi: 10.1016/j.cellsig.2012.04.004. Epub 2012 Apr 13. Cell Signal. 2012. PMID: 22522181 Free PMC article. Review.
-
Hata Y, Mori H, Tanaka A, Fujita Y, Shimomura T, Tabata T, Kinoshita K, Yamaguchi Y, Ichida F, Kominato Y, Ikeda N, Nishida N. Hata Y, et al. Int J Legal Med. 2014 Jan;128(1):105-15. doi: 10.1007/s00414-013-0853-4. Epub 2013 Apr 2. Int J Legal Med. 2014. PMID: 23546179 Review.
Cited by
-
Sala L, Yu Z, Ward-van Oostwaard D, van Veldhoven JP, Moretti A, Laugwitz KL, Mummery CL, IJzerman AP, Bellin M. Sala L, et al. EMBO Mol Med. 2016 Sep 1;8(9):1065-81. doi: 10.15252/emmm.201606260. Print 2016 Sep. EMBO Mol Med. 2016. PMID: 27470144 Free PMC article.
-
Hysteretic hERG channel gating current recorded at physiological temperature.
Jones DK. Jones DK. Sci Rep. 2022 Apr 8;12(1):5950. doi: 10.1038/s41598-022-10003-7. Sci Rep. 2022. PMID: 35396394 Free PMC article.
-
Codding SJ, Trudeau MC. Codding SJ, et al. J Gen Physiol. 2019 Apr 1;151(4):478-488. doi: 10.1085/jgp.201812129. Epub 2018 Nov 13. J Gen Physiol. 2019. PMID: 30425124 Free PMC article.
-
The S1 helix critically regulates the finely tuned gating of Kv11.1 channels.
Phan K, Ng CA, David E, Shishmarev D, Kuchel PW, Vandenberg JI, Perry MD. Phan K, et al. J Biol Chem. 2017 May 5;292(18):7688-7705. doi: 10.1074/jbc.M117.779298. Epub 2017 Mar 9. J Biol Chem. 2017. PMID: 28280240 Free PMC article.
-
Kemp JM, Whittaker DG, Venkateshappa R, Pang Z, Johal R, Sergeev V, Tibbits GF, Mirams GR, Claydon TW. Kemp JM, et al. J Gen Physiol. 2021 Oct 4;153(10):e202112923. doi: 10.1085/jgp.202112923. Epub 2021 Aug 16. J Gen Physiol. 2021. PMID: 34398210 Free PMC article.
References
Publication types
MeSH terms
Substances
LinkOut - more resources
Full Text Sources
Other Literature Sources
Miscellaneous