Rab32 is essential for maintaining functional acidocalcisomes, and for growth and infectivity of Trypanosoma cruzi - PubMed
- ️Thu Jan 01 2015
. 2015 Jun 15;128(12):2363-73.
doi: 10.1242/jcs.169466. Epub 2015 May 11.
Affiliations
- PMID: 25964650
- PMCID: PMC4487017
- DOI: 10.1242/jcs.169466
Rab32 is essential for maintaining functional acidocalcisomes, and for growth and infectivity of Trypanosoma cruzi
Sayantanee Niyogi et al. J Cell Sci. 2015.
Abstract
The contractile vacuole complex (CVC) of Trypanosoma cruzi, the etiologic agent of Chagas disease, collects and expels excess water as a mechanism of regulatory volume decrease after hyposmotic stress; it also has a role in cell shrinking after hyperosmotic stress. Here, we report that, in addition to its role in osmoregulation, the CVC of T. cruzi has a role in the biogenesis of acidocalcisomes. Expression of dominant-negative mutants of the CVC-located small GTPase Rab32 (TcCLB.506289.80) results in lower numbers of less-electron-dense acidocalcisomes, lower content of polyphosphate, lower capacity for acidocalcisome acidification and Ca(2+) uptake that is driven by the vacuolar proton pyrophosphatase and the Ca(2+)-ATPase, respectively, as well as less-infective parasites, revealing the role of this organelle in parasite infectivity. By using fluorescence, electron microscopy and electron tomography analyses, we provide further evidence of the active contact of acidocalcisomes with the CVC, indicating an active exchange of proteins between the two organelles.
Keywords: Acidocalcisome; Calcium; Contractile vacuole; Polyphosphate; Vacuolar pyrophosphatase.
© 2015. Published by The Company of Biologists Ltd.
Conflict of interest statement
Competing interests
The authors declare no competing or financial interests.
Figures
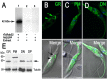
TcRab32 is geranylgeranylated in vitro. (A) Radiolabeled proteins were analyzed using SDS-PAGE on a 15% gel followed by autoradiography. Lane 1, in the presence of all reactants – rTcRab32, epimastigote extract and [H]GGPP. Lanes 2 and 3 are negative controls. The enzyme assay was performed for 30 min. A radioactive band of 42 kDa was observed, corresponding to the His-tagged protein (arrow). (B) GFP–TcRab32-expressing epimastigotes (GR) show preferential localization in the contractile vacuole (arrow) and perinuclear region (arrowhead). (C) GFP–TcRab32 prenylation-motif mutants have a cytosolic localization. (D) Mutant dominant-negative GFP–TcRab32, which mimics the GDP-bound state of the protein, has a cytosolic localization. Scale bars: 10 µm. (E) Western blot analysis of lysates from epimastigotes expressing GFP–TcRab32 (GR) or expressing the prenylation mutant (PM) or dominant negative (DN) TcRab32 using an antibody against TcRab32. Tubulin (antibody dilution 1:10,000) was used as a loading control. Arrow shows the molecular mass of GR and dominant-negative TcRab32, as well as the endogenous Rab32 in the PM lane. The prenylation mutant has a higher molecular mass.
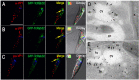
Colocalization of GFP–TcRab32 with staining of VP1 under osmotic stress. (A) There is no colocalization between VP1 (red) and GFP–TcRab32 (green), as detected with antibodies against T. brucei VP1 and GFP under isosmotic conditions. (B,C) Overlap between signals for VP1 (red) and GFP–TcRab32 (green) in the CVC occurs under hyposmotic (B) or hyperosmotic (C) stress conditions (arrows, and enlarged insets in the merged images). (D,E) Cryo-immunogold electron microscopy analysis of epimastigotes subjected to hyposmotic conditions (150 mosm/l) and incubated with rabbit anti-GFP antibodies (1:25) and mouse anti-VP1 antibodies (1:25, 12-nm gold particles, arrows) at 4°C overnight, and then treated with secondary antibodies (goat anti-mouse conjugated to 12-nm colloidal gold, arrows) and goat anti-rabbit 18-nm gold for 1 h at room temperature. The images show colocalization in the contractile vacuole (CV) bladder and the spongiome (Sp). Ac, acidocalcisome; FP, flagellar pocket; F, flagellum; K, kinetoplast. Scale bars: 10 µm (A, applies to A–C); 2 µm (D,E).
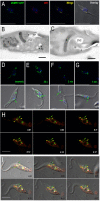
Translocation of TcVAMP7–GFP to the CVC under hyposmotic conditions. (A) Colocalization of VAMP7–GFP (green) with VP1 (red) using a polyclonal antibody against GFP and a monoclonal antibody against TcVP1, respectively. (B,C) Cryo-immunogold electron microscopy analyses using anti-GFP antibodies show predominant labeling of acidocalcisomes (Ac) under isosmotic conditions (B) and of the CVC under hyposmotic conditions (C). FP, flagellar pocket; F, flagellum; K, kinetoplast; N, nucleus. (D–G) Immunofluorescence analysis of cells imaged under isosmotic conditions (D) after 30 s (E), 2 min (F) and 5 min (G) of hyposmotic shock (150 mosm/l) using anti-GFP antibodies (green). DAPI staining labels DNA (blue). (H) Labeling of TcVAMP7–GFP-overexpressing parasites (green) with BODIPY–ceramide (red). The time indicated on each frame corresponds to 10, 30, 110, 120, 130 and 170 s (top row, left to right then bottom row, left to right). Note the dilation of the contractile vacuole bladder. (I) Overlay of differential interference contrast with the green and red channels at the same time points as those in H. In H and I, yellow arrows show acidocalcisomes, and green arrows show the localization of the CVC. Scale bars: 10 µm (A,D–G); 2 µm (B,C); 5 µm (H,I).
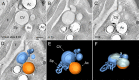
Close apposition of an acidocalcisome with the CVC, indicating a fusion event. (A–C) Virtual section (1-nm thickness) sequence of a tomogram showing the anterior region of the parasite. The CVC is represented by the central vacuole of bladder (CV) and the spongiome (Sp). Acidocalcisomes (Ac) in the neighboring region are observed in close contact with the CVC. In the left lower corner, the section number is shown. In C, it is possible to observe a close apposition between acidocalcisome and CVC membranes, which are suggestive of a fusion event (arrow) between the two organelles. (D–F) 3D models of the CVC (blue) and its close contact with an acidocalcisome (orange). (F) Tilted view of the 3D model at 45° around the x axis. Spongiome (Sp) and flagellum (‘F’) are shown. Scale bar: 200 nm.
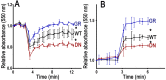
Effect of TcRab32 mutation on the parasite response to hyposmotic and hyperosmotic stress conditions. Epimastigotes were pre-incubated in isosmotic buffer for 3 min and then subjected to hyposmotic (final osmolarity=150 mosm/l) (A) or hyperosmotic (final osmolarity=650 mosm/l) (B) stress. Monitoring absorbance at 550 nm by light scattering was used to follow the relative changes in cell volume. As compared with wild-type cells (WT), cells expressing dominant-negative GFP–TcRab32 (DN) failed to fully recover their volume after hyposmotic stress and shrank less after hyperosmotic stress, whereas cells expressing GFP–TcRab32 (GR) recovered their volume faster after hyposmotic stress and shrank more after hyperosmotic stress. Values are means±s.d. of three independent experiments. Asterisks indicate statistically significant differences, P<0.05 (Bonferroni's multiple comparison ‘a posteriori’ test of one-way ANOVA), at all time points after the induction of osmotic stress (after 4 min).
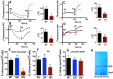
Deficient PPi-dependent H+ uptake, ATP-dependent Ca2+ uptake, acidic Ca2+ stores, short-chain polyP and PPi levels in epimastigotes expressing dominant-negative GFP–TcRab32 in comparison with wild-type and GFP–TcRab32-expressing epimastigotes. (A) Wild type (black) or epimastigotes expressing dominant-negative GFP–TcRab32 (red, DN) (5×107/ml) were added to the standard buffer containing 125 mM sucrose, 65 mM KCl, 10 mM Hepes, pH 7.2, 2.5 mM potassium phosphate, 1 mM MgCl2, to which 3 µM Acridine Orange and 40 µM digitonin were added. PPi (0.1 mM) and nigericin (1 µM, Nig) were added where indicated. (B) Epimastigotes, as above, were added to the standard buffer (in A), to which 1 µM Calcium Green-5N and 40 µM digitonin were added. ATP (1 mM) and nigericin (1 µM) were added where indicated. The bar graphs (A,B) show quantification of the initial rate of H+ (A) or Ca2+ (B) uptake after adding PPi (A) or ATP (B) post-permeabilization. Three independent experiments were used for quantification, and results are expressed as means±s.e.m. (C,D) Amastigotes (5×107/ml) loaded with Fura-2 were incubated in buffer A in the presence of 100 µM EGTA, and, where indicated, 1 µM ionomycin (Ion) or 1 µM nigericin was added. Bar graphs in C,D show quantification of the amount of Ca2+ released by the combination ionomycin-nigericin as means±s.e.m. of three independent experiments. Asterisks in A,B,D indicate that differences were significant (P<0.05). In panel C, the difference was marginal (P<0.07). Extracts from epimastigotes expressing dominant-negative GFP–TcRab32 (DN) showed ∼80% reduction in short-chain polyP levels (E) and ∼50% reduction in PPi levels (F) with no significant changes in long-chain polyP levels (G) in comparison with wild-type (WT) or GFP–TcRab32-expressing epimastigotes (GR). Values are means±s.e.m. of three independent experiments. *Differences are statistically significant as compared with respective controls, P<0.05 (0.041, DN versus GR, and 0.031, DN versus WT for E; 0.028, DN versus GR, and 0.003, DN versus WT for F) (Student's t-test). (H) Extracts of short-chain polyP produced by epimastigotes expressing GFP–TcRab32 (GR) or dominant-negative GFP–TcRab32 (DN). Two samples were resolved by using urea-PAGE and visualized using Toluidine Blue. ORG represents migration of Orange G dye. Bands corresponding to short-chain polyP of different lengths are shown as a series of bands in the lower part of the gel; these bands had lower intensities in lanes corresponding to the dominant-negative mutant (two samples) in comparison with those of cells transfected with GFP–TcRab32 (GR) and of control wild-type cells (WT) (two samples).
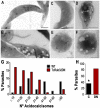
Reduction in electron-dense acidocalcisomes and a considerable increase in empty vacuoles in cells expressing dominant-negative TcRab32 in comparison with wild-type cells. (A,B) Direct transmission electron microscopy image from whole unstained and unfixed epimastigotes expressing dominant-negative GFP–TcRab32 (DN) show the presence of numerous empty vacuoles (B) in comparison with wild-type epimastigotes (A). (C–F) A similar increase in the number of empty vacuoles was observed in trypomastigotes (C,D) and amastigotes (E,F) expressing dominant-negative GFP–TcRab32 (D,F) as compared with that of wild-type cells (C,E). (G) The number of acidocalcisomes per epimastigote was counted in 70 random cells from two independent experiments, and the numeric distribution of acidocalcisomes showed that the majority of epimastigotes expressing dominant-negative TcRab32 (TcRab32DN) had <10 or between 11 and 20 electron-dense acidocalcisomes. The results in wild-type (WT) cells are similar to those reported previously for trypanosomatids. (H) An increase in the percentage of epimastigotes showing empty vacuoles. In order to quantify the phenotype of empty vacuoles, by using transmission electron microscopy, we examined 50 random wild-type parasites, ‘C’, and parasites expressing the dominant-negative protein (DN), and counted the number of parasites with empty vacuoles for each. In comparison with wild type, we found that there was a significant increase in the percentage of parasites with empty vacuoles when they expressed dominant-negative TcRab32. *Differences between control and the dominant-negative protein are statistically significant, P<0.05. Scale bars: 2 µm (A,B); 1 µm (C–F).
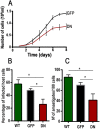
Reduced infectivity of GFP–TcRab32 mutant trypomastigotes. (A) Growth rate of epimastigotes expressing dominant-negative (DN) GFP–TcRab32 in comparison to GFP-expressing epimastigotes (GFP). Values are means±s.d. from three independent experiments. (B,C) The effect of expression of dominant-negative GFP–TcRab32 (DN) on the invasion by trypomastigotes of host cells in vitro in comparison with wild-type trypomastigotes (WT) or GFP-expressing trypomastigotes (GFP). Values are mean±s.d. (n=3). *Differences are statistically significant, P<0.05 (one way ANOVA with Bonferroni post-hoc test).
Similar articles
-
Docampo R, Jimenez V, Lander N, Li ZH, Niyogi S. Docampo R, et al. Int Rev Cell Mol Biol. 2013;305:69-113. doi: 10.1016/B978-0-12-407695-2.00002-0. Int Rev Cell Mol Biol. 2013. PMID: 23890380 Free PMC article. Review.
-
Mantilla BS, Azevedo C, Denny PW, Saiardi A, Docampo R. Mantilla BS, et al. mBio. 2021 Dec 21;12(6):e0198121. doi: 10.1128/mBio.01981-21. Epub 2021 Nov 2. mBio. 2021. PMID: 34724827 Free PMC article.
-
Rohloff P, Montalvetti A, Docampo R. Rohloff P, et al. J Biol Chem. 2004 Dec 10;279(50):52270-81. doi: 10.1074/jbc.M410372200. Epub 2004 Oct 4. J Biol Chem. 2004. PMID: 15466463
-
The role of acidocalcisomes in the stress response of Trypanosoma cruzi.
Docampo R, Jimenez V, King-Keller S, Li ZH, Moreno SN. Docampo R, et al. Adv Parasitol. 2011;75:307-24. doi: 10.1016/B978-0-12-385863-4.00014-9. Adv Parasitol. 2011. PMID: 21820562 Free PMC article.
-
A contractile vacuole complex is involved in osmoregulation in Trypanosoma cruzi.
Rohloff P, Docampo R. Rohloff P, et al. Exp Parasitol. 2008 Jan;118(1):17-24. doi: 10.1016/j.exppara.2007.04.013. Epub 2007 May 10. Exp Parasitol. 2008. PMID: 17574552 Free PMC article. Review.
Cited by
-
Potapenko E, Cordeiro CD, Huang G, Storey M, Wittwer C, Dutta AK, Jessen HJ, Starai VJ, Docampo R. Potapenko E, et al. J Biol Chem. 2018 Dec 7;293(49):19101-19112. doi: 10.1074/jbc.RA118.005884. Epub 2018 Oct 12. J Biol Chem. 2018. PMID: 30315104 Free PMC article.
-
The origin and evolution of the acidocalcisome and its interactions with other organelles.
Docampo R. Docampo R. Mol Biochem Parasitol. 2016 Sep-Oct;209(1-2):3-9. doi: 10.1016/j.molbiopara.2015.10.003. Epub 2015 Oct 30. Mol Biochem Parasitol. 2016. PMID: 26523947 Free PMC article. Review.
-
New insights into the role of acidocalcisomes in trypanosomatids.
Docampo R, Huang G. Docampo R, et al. J Eukaryot Microbiol. 2022 Nov;69(6):e12899. doi: 10.1111/jeu.12899. Epub 2022 Mar 8. J Eukaryot Microbiol. 2022. PMID: 35191563 Free PMC article. Review.
-
Evolution of the endomembrane systems of trypanosomatids - conservation and specialisation.
Venkatesh D, Boehm C, Barlow LD, Nankissoor NN, O'Reilly A, Kelly S, Dacks JB, Field MC. Venkatesh D, et al. J Cell Sci. 2017 Apr 15;130(8):1421-1434. doi: 10.1242/jcs.197640. Epub 2017 Apr 6. J Cell Sci. 2017. PMID: 28386020 Free PMC article.
-
Quantitative assessment of the nanoanatomy of the contractile vacuole complex in Trypanosoma cruzi.
Augusto I, Girard-Dias W, Schoijet A, Alonso GD, Portugal RV, de Souza W, Jimenez V, Miranda K. Augusto I, et al. Life Sci Alliance. 2024 Jul 29;7(10):e202402826. doi: 10.26508/lsa.202402826. Print 2024 Oct. Life Sci Alliance. 2024. PMID: 39074903 Free PMC article.
References
Publication types
MeSH terms
Substances
Grants and funding
LinkOut - more resources
Full Text Sources
Other Literature Sources
Medical
Miscellaneous