Hybrid zones: windows on climate change - PubMed
Review
Hybrid zones: windows on climate change
Scott A Taylor et al. Trends Ecol Evol. 2015 Jul.
Abstract
Defining the impacts of anthropogenic climate change on biodiversity and species distributions is currently a high priority. Niche models focus primarily on predicted changes in abiotic factors; however, species interactions and adaptive evolution will impact the ability of species to persist in the face of changing climate. Our review focuses on the use of hybrid zones to monitor responses of species to contemporary climate change. Monitoring hybrid zones provides insight into how range boundaries shift in response to climate change by illuminating the combined effects of species interactions and physiological sensitivity. At the same time, the semipermeable nature of species boundaries allows us to document adaptive introgression of alleles associated with response to climate change.
Keywords: adaptive introgression; distribution; gene flow; hybridization; range limits.
Copyright © 2015 Elsevier Ltd. All rights reserved.
Figures
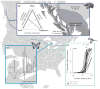
(A) Spruce hybrid zones (Picea glauca × P. engelmannii and P. glauca × P. sitchensis) [90]. The map to the right shows species distributions (indicated by dark gray (P. sitchensis), medium gray (P. engelmannii) and light gray (P. glauca)) and locations of the two hybrid zones. The triangle on the left summarizes estimated gene flow between the three species. Line width roughly corresponds to the percentage of shared alleles. Dashed lines indicate potential gene flow between two parental species and admixed individuals from the other hybrid zone. Climatic variables that are associated with each hybrid zone are indicated on the outside of the triangle. (B) Swallowtail butterfly hybrid zone (Papilio glaucus and P. canadensis) [13]. The map indicates the hybrid zone (dashed line) running east to west across Wisconsin. Lines represent the extent and direction (north or south) of species-specific trait introgression from 1998 to 2011. (C) Chickadee hybrid zone (Poecile atricapillus and P. carolinensis) [7]. Locus specific allele frequencies are plotted against distance along a linear transect (geographic clines) from historical (gray) and contemporary (black) sampling. Average shift north of 11.5 km in 10 years.
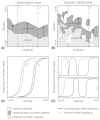
The top panels represent northward shifts of a southern species (gray) and a northern species (white) for (A) clinal and (B) mosaic hybrid zones. The gray shading represents the northern range edge of the southern species' historical (light gray, dotted line) and contemporary (dark gray, solid line) distributions. Arrows highlight the shift in the species distribution. Lines 1 and 2 represent north-south transects across the hybrid zone. The change in the northern species' allele frequency along each transect for historical (dotted) and contemporary (solid) samples are plotted for the clinal hybrid zone (C) and the mosaic hybrid zone (D). The clinal hybrid zone forms an extensive and narrow zone of contact that extends east and west. In the mosaic hybrid zone, parental forms occupy distinct habitat patches in a heterogeneous landscape and hybridization occurs across patch boundaries. The patterns of variation in allele frequency differ depending on the transect. As the range of the southern species shifts north, habitat patches alter; patches disappear, new patches form and the area of each patch changes.
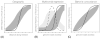
Clinal analyses for estimating introgression across a hybrid zone. Each panel depicts expected clines for a hybrid zone that is maintained by local adaptation, premating barriers that prevent the formation of hybrids, or selection against hybrids. The black lines depict a locus under selection; one that contributes to local adaptation or reproductive barriers. The gray represents the expected range of cline shapes for unlinked neutral markers. A) Classic geographic clines. B) Genomic clines modeled using multinomial regression [80, 96]. C) Genomic clines modeled using either Barton's concordance method [97], Bayesian genomic clines [98] or the log-logistic method[74].
Similar articles
-
Impacts of Niche Breadth and Dispersal Ability on Macroevolutionary Patterns.
Qiao H, Saupe EE, Soberón J, Peterson AT, Myers CE. Qiao H, et al. Am Nat. 2016 Aug;188(2):149-62. doi: 10.1086/687201. Epub 2016 May 31. Am Nat. 2016. PMID: 27420781
-
Ryan SF, Deines JM, Scriber JM, Pfrender ME, Jones SE, Emrich SJ, Hellmann JJ. Ryan SF, et al. Proc Natl Acad Sci U S A. 2018 Mar 6;115(10):E2284-E2291. doi: 10.1073/pnas.1714950115. Epub 2018 Feb 20. Proc Natl Acad Sci U S A. 2018. PMID: 29463695 Free PMC article.
-
Adaptive introgression as a resource for management and genetic conservation in a changing climate.
Hamilton JA, Miller JM. Hamilton JA, et al. Conserv Biol. 2016 Feb;30(1):33-41. doi: 10.1111/cobi.12574. Epub 2015 Oct 19. Conserv Biol. 2016. PMID: 26096581
-
Holyoak M, Heath SK. Holyoak M, et al. Integr Zool. 2016 Jan;11(1):40-59. doi: 10.1111/1749-4877.12167. Integr Zool. 2016. PMID: 26458303 Review.
-
Facilitating climate-change-induced range shifts across continental land-use barriers.
Robillard CM, Coristine LE, Soares RN, Kerr JT. Robillard CM, et al. Conserv Biol. 2015 Dec;29(6):1586-95. doi: 10.1111/cobi.12556. Epub 2015 Jul 20. Conserv Biol. 2015. PMID: 26193759 Review.
Cited by
-
Trait Variation and Spatiotemporal Dynamics across Avian Secondary Contact Zones.
Wang S, Wu L, Zhu Q, Wu J, Tang S, Zhao Y, Cheng Y, Zhang D, Qiao G, Zhang R, Lei F. Wang S, et al. Biology (Basel). 2024 Aug 22;13(8):643. doi: 10.3390/biology13080643. Biology (Basel). 2024. PMID: 39194581 Free PMC article. Review.
-
Capblancq T, Després L, Mavárez J. Capblancq T, et al. Evol Appl. 2020 Feb 7;13(6):1435-1450. doi: 10.1111/eva.12925. eCollection 2020 Jul. Evol Appl. 2020. PMID: 32684968 Free PMC article.
-
Climate change differentially alters distribution of two marten species in a hybrid zone.
Chmura HE, Olson LE, Murdoch R, Fraik AK, Jackson S, McKelvey KS, Koenig R, Pilgrim KL, DeCesare N, Schwartz MK. Chmura HE, et al. Ecol Evol. 2024 Aug 19;14(8):e70181. doi: 10.1002/ece3.70181. eCollection 2024 Aug. Ecol Evol. 2024. PMID: 39165541 Free PMC article.
-
Deacon NJ, Grossman JJ, Cavender-Bares J. Deacon NJ, et al. Ecol Evol. 2019 Jun 25;9(14):8062-8074. doi: 10.1002/ece3.5364. eCollection 2019 Jul. Ecol Evol. 2019. PMID: 31380071 Free PMC article.
-
Reticulate evolution in the Pteris fauriei group (Pteridaceae).
Chao YS, Ebihara A, Chiou WL, Tsai JM, Huang YW, Ranker TA. Chao YS, et al. Sci Rep. 2022 Jun 1;12(1):9145. doi: 10.1038/s41598-022-11390-7. Sci Rep. 2022. PMID: 35650209 Free PMC article.
References
-
- Rosenzweig C, et al. Attributing physical and biological impacts to anthropogenic climate change. Nature. 2008;453:353–357. - PubMed
-
- Lavergne S, et al. Biodiversity and climate change: integrating evolutionary and ecological responses of species and communities. Ann Rev Ecol Evol Syst. 2010;41:321–350.
-
- Quintero I, Wiens JJ. Rates of projected climate change dramatically exceed past rates of climatic niche evolution among vertebrate species. Ecol Lett. 2013;16:1095–1103. - PubMed
-
- Norberg J, et al. Eco-evolutionary responses of biodiversity to climate change. Nature Clim Change. 2012;2:747–751.
Publication types
MeSH terms
LinkOut - more resources
Full Text Sources
Other Literature Sources
Medical