Visualizing viral protein structures in cells using genetic probes for correlated light and electron microscopy - PubMed
- ️Thu Jan 01 2015
Review
Visualizing viral protein structures in cells using genetic probes for correlated light and electron microscopy
Horng D Ou et al. Methods. 2015.
Abstract
Structural studies of viral proteins most often use high-resolution techniques such as X-ray crystallography, nuclear magnetic resonance, single particle negative stain, or cryo-electron microscopy (EM) to reveal atomic interactions of soluble, homogeneous viral proteins or viral protein complexes. Once viral proteins or complexes are separated from their host's cellular environment, their natural in situ structure and details of how they interact with other cellular components may be lost. EM has been an invaluable tool in virology since its introduction in the late 1940's and subsequent application to cells in the 1950's. EM studies have expanded our knowledge of viral entry, viral replication, alteration of cellular components, and viral lysis. Most of these early studies were focused on conspicuous morphological cellular changes, because classic EM metal stains were designed to highlight classes of cellular structures rather than specific molecular structures. Much later, to identify viral proteins inducing specific structural configurations at the cellular level, immunostaining with a primary antibody followed by colloidal gold secondary antibody was employed to mark the location of specific viral proteins. This technique can suffer from artifacts in cellular ultrastructure due to compromises required to provide access to the immuno-reagents. Immunolocalization methods also require the generation of highly specific antibodies, which may not be available for every viral protein. Here we discuss new methods to visualize viral proteins and structures at high resolutions in situ using correlated light and electron microscopy (CLEM). We discuss the use of genetically encoded protein fusions that oxidize diaminobenzidine (DAB) into an osmiophilic polymer that can be visualized by EM. Detailed protocols for applying the genetically encoded photo-oxidizing protein MiniSOG to a viral protein, photo-oxidation of the fusion protein to yield DAB polymer staining, and preparation of photo-oxidized samples for TEM and serial block-face scanning EM (SBEM) for large-scale volume EM data acquisition are also presented. As an example, we discuss the recent multi-scale analysis of Adenoviral protein E4-ORF3 that reveals a new type of multi-functional polymer that disrupts multiple cellular proteins. This new capability to visualize unambiguously specific viral protein structures at high resolutions in the native cellular environment is revealing new insights into how they usurp host proteins and functions to drive pathological viral replication.
Keywords: Adenoviral proteins; E4-ORF3 polymer; Electron microscopy; MiniSOG; Photo-oxidation; Serial block face scanning electron microscopy.
Copyright © 2015 Elsevier Inc. All rights reserved.
Figures
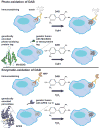
Monomeric diaminobenzidine near an oxidation source will form an osmiophilic polymer that is electron opaque in EM after osmium tetroxide staining. DAB can be photo-oxidized with certain fluorophores or through enzymatic oxidation with the addition of hydrogen peroxide. Immunostaining by antibody or genetically encoded tags can be used to position the photo-oxidizer or enzyme near the protein of interest. As an example, the figure shows how a hypothetical fibrous structure in the cytoplasm of a cell can be detected by DAB-based staining through either photo-oxidation (antibody-conjugated with eosin or a MiniSOG or a tetracysteine tag) or enzymatic oxidation (antibody-conjugated with horseradish peroxidase (HRP) or an engineered peroxidase protein APEX tag). Structural homologs of MiniSOG (PDB: 4EEP) and APEX (PDB: 3V0H) are shown.
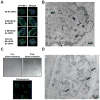
(A) U2OS cells were transfected with different MiniSOG-E4-ORF3 constructs fused at different positions and immunostained for E4-ORF3 (green). Wt-E4-ORF3 was transfected as a control. Only internal insertion of MiniSOG to E4-ORF3 displayed the correct nuclear polymer similar to Wt-E4-ORF3. DNA is stained with Hoechst. (B) U2OS cells infected with Wt Adenovirus expressing E4-ORF3 were fixed at 36 hours post infection, stained with osmium tetroxide and uranyl acetate, embedded in plastic resin, sectioned into 70–80 nm thin sections and imaged with TEM. A sample image shows many distinctive cellular and viral structures in an Adenovirus infected cell: the paracrystalline lattice (solid arrow), round, grainy viral replication domains (dashed arrow), and electron-dense particles (double arrowhead). Strikingly, no polymer structure is observed that resembles the E4-ORF3 immunofluorescence image. (C) U2OS cells infected with Adenovirus expressing MiniSOG-E4-ORF3 were photo-oxidized for 6 minutes in DAB buffer. MiniSOG-E4-ORF3 was not visible in the bright field image before photo-oxidation (left panel), but highly visible after photo-oxidation (right panel). Lower panel shows the fluorescence image of MiniSOG-E4-ORF3. (D) Thin sectioned TEM image of a photo-oxidized U2OS cell infected with Adenovirus expressing MiniSOG-E4-ORF3. The E4-ORF3 polymer (arrow) is highly visible in comparison with a conventionally stained infected U2OS cell in (B) due to DAB polymer precipitation along the E4-ORF3 polymer.
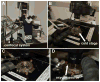
(A) A Leica/SP II confocal microscope for photo-oxidation. (B–C) A brass cold stage connected to a water cooler to maintain sample at 4°C. (D) A tube delivers a stream of oxygen above the sample during photo-oxidation to maintain an oxygenated environment to facilitate photo-oxidation of DAB.
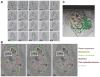
(A) U2OS cells infected with Adenovirus expressing MiniSOG-E4-ORF3 (dark structures) were fixed at 36 hours post infection, photo-oxidized and prepared for serial block face SEM imaging (SBEM). An array of individual images is shown. Each image plane was recorded at 60 nm intervals, and the entire cell was recorded using 150 image planes. (B) Segmentation of E4-ORF3 and other cellular structures in a cell from the SBEM volume from (A). Cell membrane (purple), nuclear membrane (yellow), E4-ORF3 (white), viral replication domains (red), nucleolus (blue), and mitochondria (green) were manually segmented in IMOD. Three sample slices are shown here. White box marks the tracing of E4-ORF3 polymer. (C) Rendering of the segmented structures from (B) showing E4-ORF3 polymer form a network that circumnavigates around nucleoli and the viral replication domains. All rendered surfaces are labeled in the same color as (B).
Similar articles
-
Shu X, Lev-Ram V, Deerinck TJ, Qi Y, Ramko EB, Davidson MW, Jin Y, Ellisman MH, Tsien RY. Shu X, et al. PLoS Biol. 2011 Apr;9(4):e1001041. doi: 10.1371/journal.pbio.1001041. Epub 2011 Apr 5. PLoS Biol. 2011. PMID: 21483721 Free PMC article.
-
A workflow for 3D-CLEM investigating liver tissue.
Kremer A, VAN Hamme E, Bonnardel J, Borghgraef P, GuÉrin CJ, Guilliams M, Lippens S. Kremer A, et al. J Microsc. 2021 Mar;281(3):231-242. doi: 10.1111/jmi.12967. Epub 2020 Oct 27. J Microsc. 2021. PMID: 33034376
-
Identification of PSD-95 in the Postsynaptic Density Using MiniSOG and EM Tomography.
Chen X, Winters C, Crocker V, Lazarou M, Sousa AA, Leapman RD, Reese TS. Chen X, et al. Front Neuroanat. 2018 Dec 7;12:107. doi: 10.3389/fnana.2018.00107. eCollection 2018. Front Neuroanat. 2018. PMID: 30581381 Free PMC article.
-
Romero-Brey I, Bartenschlager R. Romero-Brey I, et al. Viruses. 2015 Dec 3;7(12):6316-45. doi: 10.3390/v7122940. Viruses. 2015. PMID: 26633469 Free PMC article. Review.
-
Determining the Crystal Structure of TRPV6.
Saotome K, Singh AK, Sobolevsky AI. Saotome K, et al. In: Kozak JA, Putney JW Jr, editors. Calcium Entry Channels in Non-Excitable Cells. Boca Raton (FL): CRC Press/Taylor & Francis; 2018. Chapter 14. In: Kozak JA, Putney JW Jr, editors. Calcium Entry Channels in Non-Excitable Cells. Boca Raton (FL): CRC Press/Taylor & Francis; 2018. Chapter 14. PMID: 30299652 Free Books & Documents. Review.
Cited by
-
An optogenetic toolbox of LOV-based photosensitizers for light-driven killing of bacteria.
Endres S, Wingen M, Torra J, Ruiz-González R, Polen T, Bosio G, Bitzenhofer NL, Hilgers F, Gensch T, Nonell S, Jaeger KE, Drepper T. Endres S, et al. Sci Rep. 2018 Oct 9;8(1):15021. doi: 10.1038/s41598-018-33291-4. Sci Rep. 2018. PMID: 30301917 Free PMC article.
-
Rational Design of Bioavailable Photosensitizers for Manipulation and Imaging of Biological Systems.
Binns TC, Ayala AX, Grimm JB, Tkachuk AN, Castillon GA, Phan S, Zhang L, Brown TA, Liu Z, Adams SR, Ellisman MH, Koyama M, Lavis LD. Binns TC, et al. Cell Chem Biol. 2020 Aug 20;27(8):1063-1072.e7. doi: 10.1016/j.chembiol.2020.07.001. Epub 2020 Jul 21. Cell Chem Biol. 2020. PMID: 32698018 Free PMC article.
-
ChromEMT: Visualizing 3D chromatin structure and compaction in interphase and mitotic cells.
Ou HD, Phan S, Deerinck TJ, Thor A, Ellisman MH, O'Shea CC. Ou HD, et al. Science. 2017 Jul 28;357(6349):eaag0025. doi: 10.1126/science.aag0025. Science. 2017. PMID: 28751582 Free PMC article.
-
Shedding light on biology of bacterial cells.
Schneider JP, Basler M. Schneider JP, et al. Philos Trans R Soc Lond B Biol Sci. 2016 Nov 5;371(1707):20150499. doi: 10.1098/rstb.2015.0499. Philos Trans R Soc Lond B Biol Sci. 2016. PMID: 27672150 Free PMC article. Review.
-
Chromothripsis drives the evolution of gene amplification in cancer.
Shoshani O, Brunner SF, Yaeger R, Ly P, Nechemia-Arbely Y, Kim DH, Fang R, Castillon GA, Yu M, Li JSZ, Sun Y, Ellisman MH, Ren B, Campbell PJ, Cleveland DW. Shoshani O, et al. Nature. 2021 Mar;591(7848):137-141. doi: 10.1038/s41586-020-03064-z. Epub 2020 Dec 23. Nature. 2021. PMID: 33361815 Free PMC article.
References
Publication types
MeSH terms
Substances
Grants and funding
LinkOut - more resources
Full Text Sources
Other Literature Sources
Research Materials