Kinetic discrimination of self/non-self RNA by the ATPase activity of RIG-I and MDA5 - PubMed
- ️Thu Jan 01 2015
Kinetic discrimination of self/non-self RNA by the ATPase activity of RIG-I and MDA5
Jade Louber et al. BMC Biol. 2015.
Abstract
Background: The cytoplasmic RIG-like receptors are responsible for the early detection of viruses and other intracellular microbes by activating the innate immune response mediated by type I interferons (IFNs). RIG-I and MDA5 detect virus-specific RNA motifs with short 5'-tri/diphosphorylated, blunt-end double-stranded RNA (dsRNA) and >0.5-2 kb long dsRNA as canonical agonists, respectively. However, in vitro, they can bind to many RNA species, while in cells there is an activation threshold. As SF2 helicase/ATPase family members, ATP hydrolysis is dependent on co-operative RNA and ATP binding. Whereas simultaneous ATP and cognate RNA binding is sufficient to activate RIG-I by releasing autoinhibition of the signaling domains, the physiological role of the ATPase activity of RIG-I and MDA5 remains controversial.
Results: A cross-analysis of a rationally designed panel of RNA binding and ATPase mutants and truncated receptors, using type I IFN promoter activation as readout, allows us to refine our understanding of the structure-function relationships of RIG-I and MDA5. RNA activation of RIG-I depends on multiple critical RNA binding sites in its helicase domain as confirmed by functional evidence using novel mutations. We found that RIG-I or MDA5 mutants with low ATP hydrolysis activity exhibit constitutive activity but this was fully reverted when associated with mutations preventing RNA binding to the helicase domain. We propose that the turnover kinetics of the ATPase domain enables the discrimination of self/non-self RNA by both RIG-I and MDA5. Non-cognate, possibly self, RNA binding would lead to fast ATP turnover and RNA disassociation and thus insufficient time for the caspase activation and recruitment domains (CARDs) to promote downstream signaling, whereas tighter cognate RNA binding provides a longer time window for downstream events to be engaged.
Conclusions: The exquisite fine-tuning of RIG-I and MDA5 RNA-dependent ATPase activity coupled to CARD release allows a robust IFN response from a minor subset of non-self RNAs within a sea of cellular self RNAs. This avoids the eventuality of deleterious autoimmunity effects as have been recently described to arise from natural gain-of-function alleles of RIG-I and MDA5.
Figures
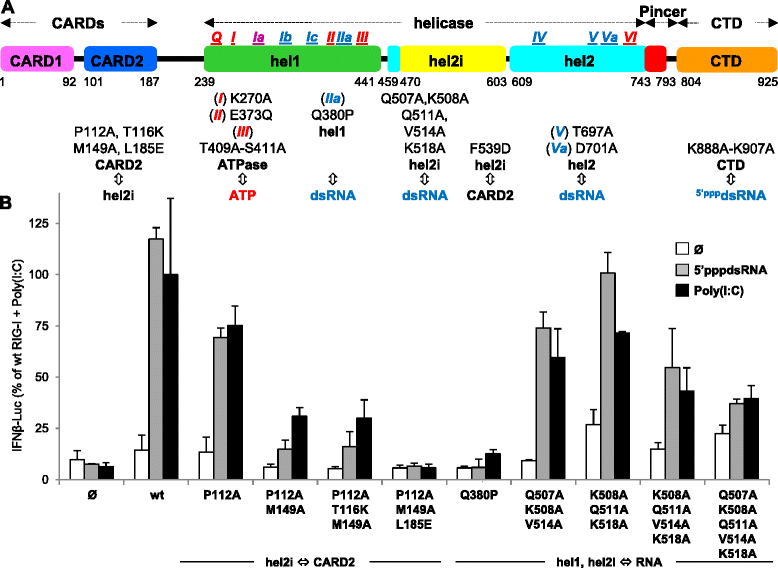
Refinement of RIG-I hel residues involved in hel2i-CARD2 interaction and RNA binding as assessed by RIG-I ability to activate the human IFNβ-promoter in Huh7.5 cells. a Modular domain organization of RIG-I and location of amino acid substitution investigated in this study. hel motifs involved in ATP binding or RNA binding are represented in blue and red, respectively, and in violet when involved in both of them. b Functional investigation in Huh7.5 cells of hel2i residues contacting CARD2 F539 residue in RIG-I auto-repressed form and hel1/hel2i residues involved in RNA binding without RNA (white columns), or stimulated with 5′pppdsRNA (grey columns) or poly(I:C) (black columns). Data (mean +/− SD of three independent replicates, and the experiment was performed twice with similar results) were expressed in fold response of wt RIG-I with poly(I:C) set up as 100 % activity. See Additional file 2 for protein expression levels of each construct analyzed by western blot. CARD: caspase activation and recruitment domain; hel: helicase
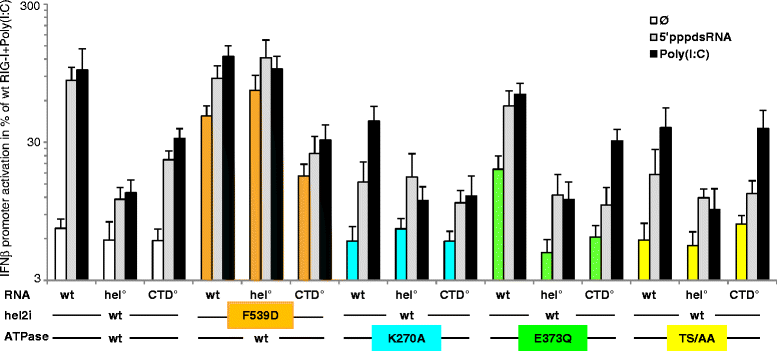
Interplay between the ATPase activity and RNA binding sites of RIG-I to elicit a signal. The ability of wt, hel2i F539D, ATPase E373Q, K270A and T409A/S411A (TS/AA) mutants RIG-I harboring or not mutations that loosen the RNA binding sites on the hel (T697A/E702A, hel°) or CTD (K888A/K907A, CTD°) domain were tested for their ability to activate the human IFNβ promoter. Note lower signalling ability of the F539D-CTD° mutant correlates with its lower expression as determined by western blot (see Additional file 2). The activity of F539D and E373Q was constitutive at P <0.0025 and P <0.01, respectively. All ATPase variants with intact hel and CTD domains significantly responded to both 5′pppdsRNA and poly(I:C) with the possible exception of TS/AA variant stimulated by the 5′pppdsRNA (P <0.05 to <0.005 range, in comparison with the signal without exogenous dsRNA). The wt, K270A, E373Q and TS/AA CTD° variants lost their response to 5′pppdsRNA (P <0.025 to <0.01 range in comparison with intact CTD counterparts), and also to the poly(I:C) in the case of K270A mutant (P <0.01). E373Q-hel° was inactive (P <0.001 versus 373Q) as were all hel° constructs (P <0.05 to <0.025 range versus counterparts with intact hel domain). The constitutive activity of the F539D mutant (P <0.0025 versus wt) remained unaffected when associated to either CTD° or hel° if the lower protein expression level of F539D-CTD° construct is taken into account. The significant response of F539D construct to both dsRNAs (P <0.05 and P <0.01 versus no RNA) was abolished if associated with hel° or CTD°. Note the log scale display of the IFNβ activation because values were within a large range. See Additional file 2 for protein expression levels of each construct analyzed by western blot. CTD: C-terminal domain; hel: helicase
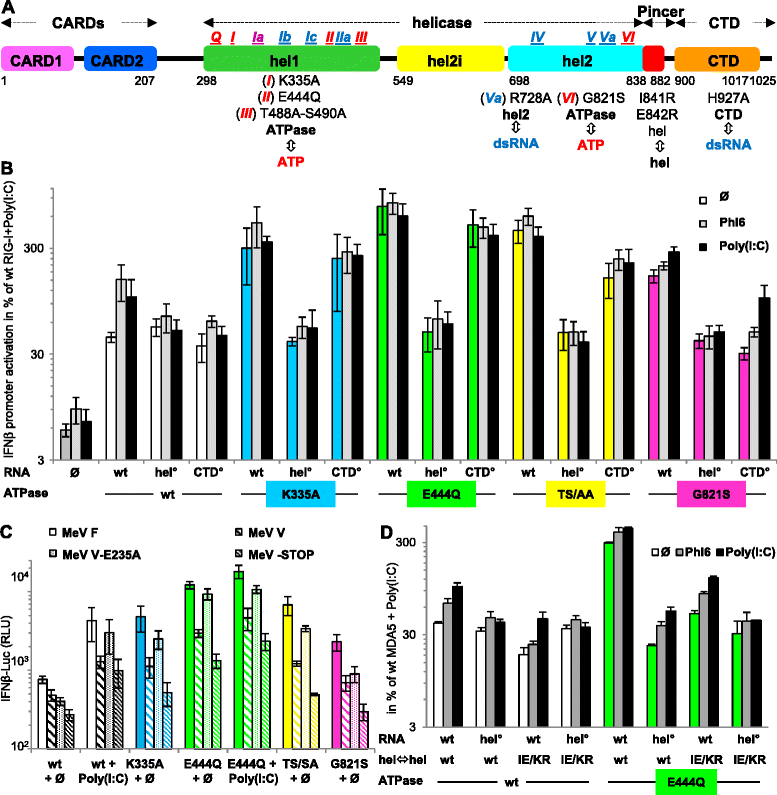
Interplay between the ATPase activity and RNA binding sites of MDA5 to elicit a signal. a Schematic domain organization of human MDA5. b Ability of MDA5 ATPase mutants to activate the IFNβ promoter in Huh7.5 cells. Expression of MDA5 activates the IFN promoter (P <0.0005). Compared to wt, ATPase variants with intact RNA binding sites exhibit a constitutive activity (P <0.05 to <0.0025). In contrast, the wt-CTD° and wt-hel° constructs, which have an altered RNA binding site due to R728A and H927A substitution, respectively, do not respond to both dsRNAs. The association of CTD° reduces the constitutive activity of only G821S (P <0.0025), with G821S-CTD° exhibiting a residual response to both dsRNAs (Phi6, P <0.025, poly(I:C), P <0.0025). Hel° inhibits both constitutive and RNA-dependent activities (P <0.05 to <0.005) down to the background activation level of IFNβ promoter observed with wt MDA5 (P <0.01 to <0.0025). c Inhibition of constitutive activity of every ATPase mutant by MeV V (P <0.025 to <0.0005 versus MeV F control) and MeV V-STOP (P <0.01 to <0.0005), but not by MeV V-E235A (P <0.05 to <0.0005). MeV V-STOP had higher inhibitory effect than MeV V (P <0.05 to <0.0005). In the presence of poly(I:C), the profile of inhibition of wt and E444Q MDA5 activity by MeV V and V-STOP was similar (MeV V or V-STOP versus F, P <0.05 to <0.005 and V-E235A versus V or V-STOP, P <0.05 to <0.0005). In panel (C), E444Q displayed the strongest constitutive activity (P <0.025 to <0.005), and K335A, TS/AA and G821S shared a similar constitutive activity. d IE/KR substitution alone significantly reduced observed responses of all constructs (P <0.05 to <0.0005 versus wt). As observed in panel (B) the introduction of hel° abolished both the response to dsRNA and constitutive activity of MDA5 variants (P <0.01 to <0.0005). Upon stimulation with Phi6 and poly(I:C), both wt and E444Q MDA5 with IE/KR substitution retained a significant activity (P <0.0025, P <0.01, P <0.0025 and P <0.0005, respectively), with a significantly higher response to poly(I:C) than to Phi6 (P <0.0125 and P <0.0025). See Additional file 2 for protein expression levels of each construct analyzed by western blot. CTD: C-terminal domain; hel: helicase; MeV: measles virus
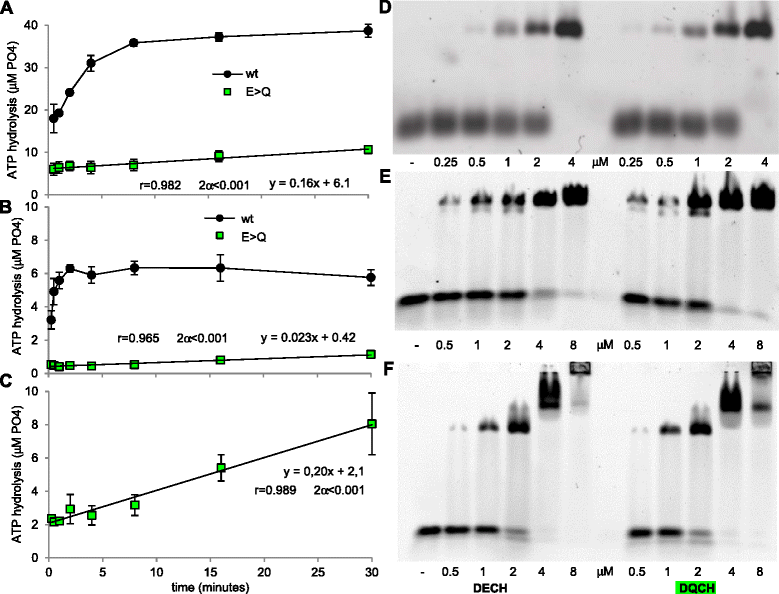
DECH/DQCH mutation in ATPase domain of a dRIG-I and b chMDA5 results in much slower ATP hydrolysis ability without affecting RNA binding and ablation of CARDs from chMDA5 DECH/DQCH mutant (hel-CTD construct), enhancing the c ATP hydrolysis rate. The hydrolysis of ATP by all three DECH/DQCH mutants increased linearly over 30 minutes as shown by equations and correlation analysis. ATP hydrolysis assay was performed by incubating a 0.5 μM RIG-I, 2 μM 12dsRNA, and b, c 2 mM ATP or 8 μM MDA5, 32 μM 24dsRNA, 2 mM ATP. d, e, f Representative EMSA showing the ability of wt (DECH) and DQCH proteins to bind to dsRNA. Each lane contains 40 ng cy3 labelled 12dsRNA (EMSA of duck RIG-I), 24dsRNA (EMSA of chicken MDA5), and increasing the concentration of d dRIG-I or e chMDA5 or f chMDA5 hel-CTD concentrations (0.25–8 μM). CARD: caspase activation and recruitment domain; CTD: C-terminal domain; EMSA: electrophoretic mobility shift assay; hel: helicase
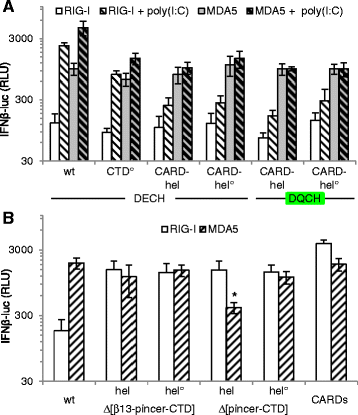
CTD deletion of both RIG-I and MDA5 locked them in an auto-repressed form which is alleviated by deletion of the pincer domain in the case of RIG-I. a CARD-hel, RIG-I 1–797 and MDA5 1–895, and CARD-hel° constructs and their DQCH mutant display only background activity similar to their full-length (wt) counterparts and do not respond to poly(I:C). Note by comparison the significant albeit reduced response to poly(I:C) of full-length RIG-I and MDA5 with CTD° unable to bind RNA (P <0.025 for both) when compared to CTD° without poly(I:C), and P <0.0025 and P <0.0125 when compared to wt constructs + poly(I:C), respectively, consistently with data shown in Figs. 2 and 3. b Further deletion of the pincer domain alone, RIG-I 1–744 quoted Δ[pincer-CTD], or together with deletion of the last hel2 βsheet, RIG-I 1–734 quoted Δ[β13pincer-CTD], results in constitutive activity of RIG-I (P <0.025 and below). In contrast, their MDA5 counterparts MDA5 1–836 quoted Δ[pincer-CTD] and MDA5 1–824 quoted Δ[β13pincer-CTD], shows no enhancement over the background activity exhibited by MDA5 hel and hel° constructs (P >0.05 and above). Free CARDs from both RIG-I (aa 1–228) and MDA5 (aa 1–294) are constitutively active (P <0.01 for both). Note that the lower basal activity (*) of MDA5 Δ[pincer-CTD] construct is associated with a lower expression level. CARD: caspase activation and recruitment domain; CTD: C-terminal domain; hel: helicase
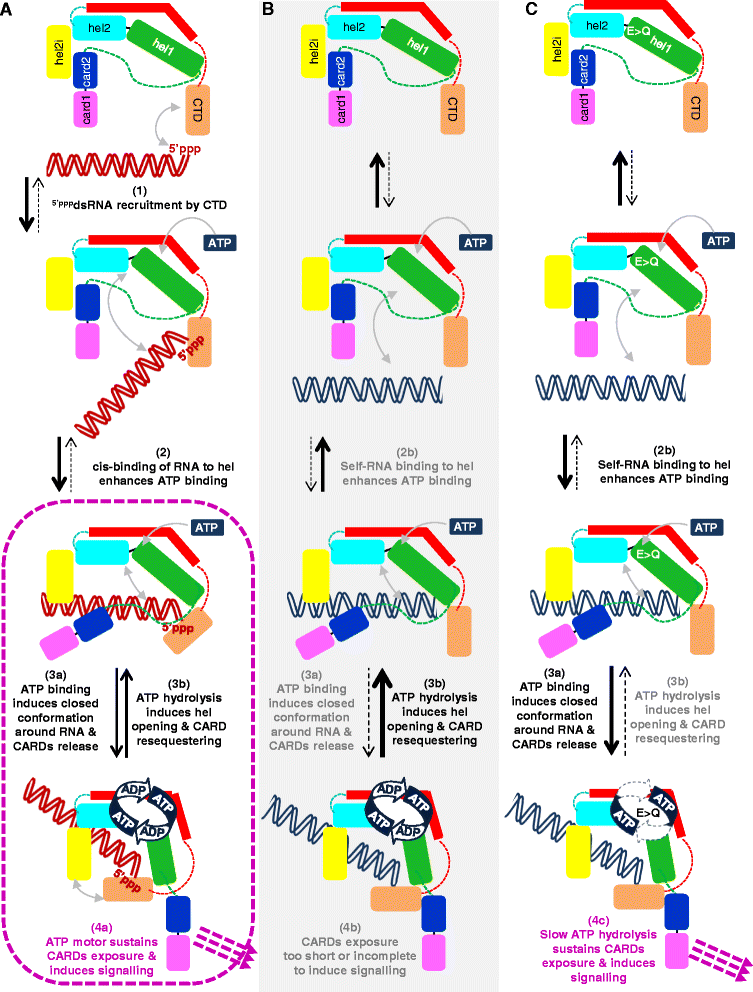
Model of ATPase-mediated discrimination of non-self RNA by RIG-I. a Activation model of wt RIG-I. In resting state, RIG-I is in an auto-repressed form with CARD2 binding to hel2i, which prevents RNA binding to the hel domain. Upon a viral infection, RIG-I CTD selectively catches non-self 5′pppdsRNA (1). This favors the cis-binding of 5′pppdsRNA to the hel domain that allows/enhances ATP binding by allosteric effect (2). ATP binding promotes RIG-I folding into closed state with release of CARD2 away from hel2i and exposure of the CARDs (3a). ATP hydrolysis causes ADP+Pi release, hel opening, allosteric weakening of the RNA to hel interaction and CARD resequestration (3b). Because remaining bound to CTD by its 5′pppdsRNA end, RNA rebinding to hel in cis is favored (3a), which resumes another cycle of ATP fixation, CARD release and ATP hydrolysis (3a and 3b). Rapid repetition of RNA/hel association/dissociation powered by the ATPase motor with on/off cycling of CARD exposure (4a) ensures a sustained level of activated RIG-I molecules per time unit above the critical threshold required for effective signalling. b A fortuitous binding of self RNA to hel (2b) induces ATP fixation, closed conformation and ATP hydrolysis that irreversibly evicts the RNA from hel, because it lacks CTD anchoring. Consequently, the CARDs are exposed too transiently, if ever, to reach the threshold number of transduction units per time unit required for signalling (4b). c Model of the constitutive activity of RIG-I-E373Q. Surrounding (self) RNA serendipitously binds to hel (2b), allows ATP binding with eviction of CARD2 from hel2i (3a). The slow ATP hydrolysis refrains the eviction of the RNA from the hel and CARD2 resequestering (step 3b is very slow). Consequently, a sustained level of activated RIG-I-E373Q molecules with their CARDs exposed accumulate above the threshold level required for IFNβ promoter activation (4c). CARD: caspase activation and recruitment domain; CTD: C-terminal domain; hel: helicase
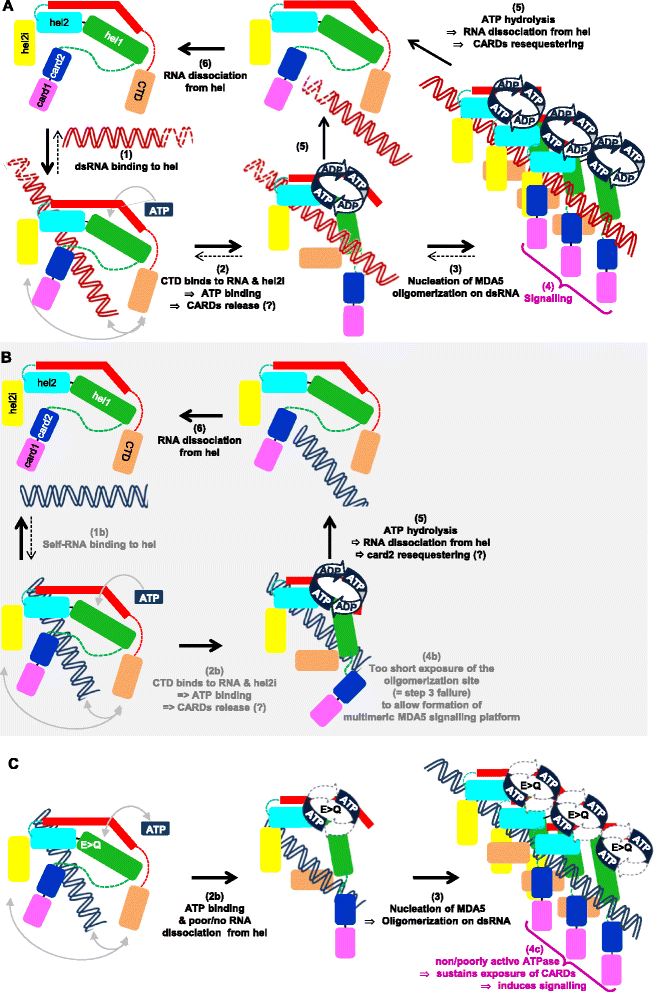
Model of ATPase-mediated discrimination of non-self RNA by MDA5. a Activation model of wt MDA5. In resting state, MDA5 is in an auto-repressed form with, by analogy with RIG-I, CARD(s) binding to hel that prevents direct access of any RNA to the hel domain. Upon encountering, a viral dsRNA binds to MDA5 hel (2). CTD binds to RNA and hel2i and induces CARD release and exposure of the self-oligomerization domain. This nucleates the binding of another MDA5 molecule on the same RNA (3) and so on so as to constitute a multivalent CARD signalling platform (4). The binding of dsRNA to the hel also allosterically favours ATP binding to the ATPase motor (2). This drives fast ATP hydrolysis (5) with allosteric release of the dsRNA from the hel domain of MDA5, disassembly of the oligomer into monomers that returns back to their auto-repressed state. b A serendipitous binding to cellular (self) RNA (1b) can induce ATP binding (2b) but the self RNA is evicted before any recruitment of another MDA5 can occur (that is, step 5 is prevalent over step 3). Consequently, there is no signal elicitation (4b). c Model of the constitutive activity of ATPase-deficient MDA5. MDA5-E444Q serendipitously binds to surrounding cellular (self) RNA via its hel domain (step 1b, not shown, see panel b) with binding of CTD to RNA and hel2i and exposure of both CARDs and oligomerization site. Because of the poor efficiency of its ATPase motor, step 5 cannot occur, the illegitimate self RNA cannot be expelled from the hel domain and multiple MDA5 in active state assemble into a long-lived multivalent platform (step 3) that keeps transducing the downstream signal (4c). Note that the multivalent platform may be made of MDA5 subunits bound to different short stretches of self RNA. CARD: caspase activation and recruitment domain; CTD: C-terminal domain; hel: helicase
Similar articles
-
RIG-I ATPase activity and discrimination of self-RNA versus non-self-RNA.
Anchisi S, Guerra J, Garcin D. Anchisi S, et al. mBio. 2015 Mar 3;6(2):e02349. doi: 10.1128/mBio.02349-14. mBio. 2015. PMID: 25736886 Free PMC article.
-
Structural Analysis of dsRNA Binding to Anti-viral Pattern Recognition Receptors LGP2 and MDA5.
Uchikawa E, Lethier M, Malet H, Brunel J, Gerlier D, Cusack S. Uchikawa E, et al. Mol Cell. 2016 May 19;62(4):586-602. doi: 10.1016/j.molcel.2016.04.021. Mol Cell. 2016. PMID: 27203181 Free PMC article.
-
Structural basis of RNA recognition and activation by innate immune receptor RIG-I.
Jiang F, Ramanathan A, Miller MT, Tang GQ, Gale M Jr, Patel SS, Marcotrigiano J. Jiang F, et al. Nature. 2011 Sep 25;479(7373):423-7. doi: 10.1038/nature10537. Nature. 2011. PMID: 21947008 Free PMC article.
-
Proofreading mechanisms of the innate immune receptor RIG-I: distinguishing self and viral RNA.
Solotchi M, Patel SS. Solotchi M, et al. Biochem Soc Trans. 2024 Jun 26;52(3):1131-1148. doi: 10.1042/BST20230724. Biochem Soc Trans. 2024. PMID: 38884803 Free PMC article. Review.
-
Structural insights into RNA recognition and activation of RIG-I-like receptors.
Leung DW, Amarasinghe GK. Leung DW, et al. Curr Opin Struct Biol. 2012 Jun;22(3):297-303. doi: 10.1016/j.sbi.2012.03.011. Epub 2012 May 2. Curr Opin Struct Biol. 2012. PMID: 22560447 Free PMC article. Review.
Cited by
-
RNA binding activates RIG-I by releasing an autorepressed signaling domain.
Dickey TH, Song B, Pyle AM. Dickey TH, et al. Sci Adv. 2019 Oct 2;5(10):eaax3641. doi: 10.1126/sciadv.aax3641. eCollection 2019 Oct. Sci Adv. 2019. PMID: 31616790 Free PMC article.
-
Cellular origins of dsRNA, their recognition and consequences.
Chen YG, Hur S. Chen YG, et al. Nat Rev Mol Cell Biol. 2022 Apr;23(4):286-301. doi: 10.1038/s41580-021-00430-1. Epub 2021 Nov 23. Nat Rev Mol Cell Biol. 2022. PMID: 34815573 Free PMC article. Review.
-
Sikorska J, Hou Y, Chiurazzi P, Siu T, Baltus GA, Sheth P, McLaren DG, Truong Q, Parish CA, Wyss DF. Sikorska J, et al. Nucleic Acids Res. 2023 Sep 22;51(17):9356-9368. doi: 10.1093/nar/gkad606. Nucleic Acids Res. 2023. PMID: 37486777 Free PMC article.
-
Durbin AF, Wang C, Marcotrigiano J, Gehrke L. Durbin AF, et al. mBio. 2016 Sep 20;7(5):e00833-16. doi: 10.1128/mBio.00833-16. mBio. 2016. PMID: 27651356 Free PMC article.
-
Comparative Structure and Function Analysis of the RIG-I-Like Receptors: RIG-I and MDA5.
Brisse M, Ly H. Brisse M, et al. Front Immunol. 2019 Jul 17;10:1586. doi: 10.3389/fimmu.2019.01586. eCollection 2019. Front Immunol. 2019. PMID: 31379819 Free PMC article. Review.
References
Publication types
MeSH terms
Substances
LinkOut - more resources
Full Text Sources
Other Literature Sources
Research Materials
Miscellaneous