Tectonic control on the persistence of glacially sculpted topography - PubMed
- ️Thu Jan 01 2015
Tectonic control on the persistence of glacially sculpted topography
Günther Prasicek et al. Nat Commun. 2015.
Abstract
One of the most fundamental insights for understanding how landscapes evolve is based on determining the extent to which topography was shaped by glaciers or by rivers. More than 10(4) years after the last major glaciation the topography of mountain ranges worldwide remains dominated by characteristic glacial landforms such as U-shaped valleys, but an understanding of the persistence of such landforms is lacking. Here we use digital topographic data to analyse valley shapes at sites worldwide to demonstrate that the persistence of U-shaped valleys is controlled by the erosional response to tectonic forcing. Our findings indicate that glacial topography in Earth's most rapidly uplifting mountain ranges is rapidly replaced by fluvial topography and hence valley forms do not reflect the cumulative action of multiple glacial periods, implying that the classic physiographic signature of glaciated landscapes is best expressed in, and indeed limited by, the extent of relatively low-uplift terrain.
Figures
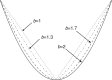
Power laws of the form y=axb fit to valley flanks with the exponent b depicting their shape. b=1 represents the straight valley flanks of a V-shaped valley and greater exponents are indicative of progressively more U-shaped valleys.
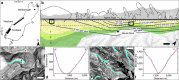
(a) Location of the main study area in Westland and the two reference study areas, Marlborough (fluvial) and Fiordland (glacial) on New Zealand's South Island. (b) Extent of Westland study area (dashed rectangular outline), LGM extent (dotted outline), modern coastline (grey shading), rock uplift rates (solid contour lines), geological map showing metamorphic grade of Alpine schist south of the Alpine Fault, Garnet-Oligoclase zone (1), Biotite zone (2), and Chlorite zone (3) (ref. 24), plotted on a digital terrain model. Bold black rectangles outline the extents of subfigures c and e. Scale bar, 10-km wide. (c) Shaded relief map and an example cross-section (dashed line; X–X′) for strongly glacial, low-uplift terrain in the Westland study area. Scale bar, 1-km wide. (d) Example cross-section (X–X′; solid line) and fitted power law (dashed line, b=1.95). (e) Shaded relief map and example cross-section (dashed line; Y–Y′) of strongly fluvial, high-uplift terrain in the Westland study area. Black polygons delimit current ice cover and are not included in analysis. Scale bar, 1-km wide. (f) Example cross-section (Y–Y'; solid line) and fitted power law (dashed line, b=0.97). Contour spacing in c and e is 500 m, cyan lines show flow path cells for valley cross-section extraction.
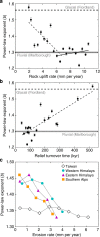
(a) Mean power-law exponents for 20 rock uplift bins from Westland (bin size=0.45 mm per year). Regression lines fitted separately to data with a rock uplift rate <6 mm per year (dashed line, y=−0.043 (±0.002)x+1.569(±0.011), R2=0.54, root-mean-squared error (r.m.s.e.)=0.06, P<0.01) and >6 mm per year (solid line, y=0.007 (±0.002)x+1.236(±0.014), R2=0.08, r.m.s.e.=0.04, P=0.4). Reference exponents: fluvial from Marlborough (1.28; solid grey line) and glacial from Fiordland (1.54; dashed grey line). Error bars indicate±1 s.e. See Supplementary Discussion and Supplementary Fig. 1 for further information on data distribution and statistics. (b) Mean power-law exponents of 20 rock uplift bins from Westland plotted against relief turnover time. Turnover time is defined as relief/erosion rate and we assume rock uplift rates equal erosion rates. Power-law exponent increases with turnover time as y=0.001(±<0.001)x+1.251(±<0.001), R2=0.67, r.m.s.e.=0.05, P<0.01. (c) Power-law exponents plotted against erosion rates for Earth's most rapidly uplifting and eroding but previously glaciated landscapes and one landscape with little glacial modification (Taiwan). A gridded erosion rate data set derived from thermochronology that is common to all four regions is used as a rock uplift rate proxy. For study area maps, see Supplementary Figs 3–5.
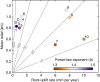
(a) Rock uplift plotted against average relief of some of Earth's major mountain ranges: Washington Cascades, WA, USA (1); Coast Mountains, BC, Canada (2); Fiordland, New Zealand (3); Greater Caucasus, Georgia/Russia (4); Northern Patagonian Andes, Chile (5); European Alps, Austria/Italy/Switzerland (6); Central Range, Taiwan (7); Eastern Himalaya, China (8); Western Himalaya, Pakistan (9); and High-uplift Westland, New Zealand (10). Contours indicate time (kyr) necessary to renew 20% of the relief, the average fraction of relief turnover time required to transform a U-shaped into a V-shaped valley in Westland. The minimum, mean and maximum durations of the last four interglacial periods are 20, 22 and 26 kyr, respectively, indicating that transformation from glacial to fluvial landscape morphology can occur during a single interglacial period for comparable or lower contour values. Power-law exponents indicate the degree of glacial imprint on topography. See Supplementary Fig. 6 for study area maps.
Similar articles
-
Multi-scale curvature for automated identification of glaciated mountain landscapes.
Prasicek G, Otto JC, Montgomery DR, Schrott L. Prasicek G, et al. Geomorphology (Amst). 2014 Mar 15;209(100):53-65. doi: 10.1016/j.geomorph.2013.11.026. Geomorphology (Amst). 2014. PMID: 24748703 Free PMC article.
-
Glacial effects limiting mountain height.
Egholm DL, Nielsen SB, Pedersen VK, Lesemann JE. Egholm DL, et al. Nature. 2009 Aug 13;460(7257):884-7. doi: 10.1038/nature08263. Nature. 2009. PMID: 19675651
-
Thermochronometry reveals headward propagation of erosion in an alpine landscape.
Shuster DL, Cuffey KM, Sanders JW, Balco G. Shuster DL, et al. Science. 2011 Apr 1;332(6025):84-8. doi: 10.1126/science.1198401. Science. 2011. PMID: 21454785
-
Shafer AB, Cullingham CI, Côté SD, Coltman DW. Shafer AB, et al. Mol Ecol. 2010 Nov;19(21):4589-621. doi: 10.1111/j.1365-294X.2010.04828.x. Epub 2010 Sep 17. Mol Ecol. 2010. PMID: 20849561 Review.
-
Birth of a biome: insights into the assembly and maintenance of the Australian arid zone biota.
Byrne M, Yeates DK, Joseph L, Kearney M, Bowler J, Williams MA, Cooper S, Donnellan SC, Keogh JS, Leys R, Melville J, Murphy DJ, Porch N, Wyrwoll KH. Byrne M, et al. Mol Ecol. 2008 Oct;17(20):4398-417. doi: 10.1111/j.1365-294X.2008.03899.x. Epub 2008 Aug 27. Mol Ecol. 2008. PMID: 18761619 Review.
Cited by
-
Bandou D, Schlunegger F, Kissling E, Marti U, Schwenk M, Schläfli P, Douillet G, Mair D. Bandou D, et al. Sci Rep. 2022 Jan 27;12(1):1441. doi: 10.1038/s41598-022-04830-x. Sci Rep. 2022. PMID: 35087080 Free PMC article.
-
Glacial Steady State Topography Controlled by the Coupled Influence of Tectonics and Climate.
Prasicek G, Herman F, Robl J, Braun J. Prasicek G, et al. J Geophys Res Earth Surf. 2018 Jun;123(6):1344-1362. doi: 10.1029/2017JF004559. Epub 2018 Jun 19. J Geophys Res Earth Surf. 2018. PMID: 30069424 Free PMC article.
References
-
- Agassiz L. Études sur les glaciers Jent et Gassmann (1840).
-
- Davis W. M. Glacial erosion in France, Switzerland and Norway. Proc. Boston Soc. Nat. Hist. 29, 273–322 (1900).
-
- Brocklehurst S. H. & Whipple K. X. Hypsometry of glaciated landscapes. Earth Surf. Process. Landforms 29, 907–926 (2004).
-
- Larsen I. J. & Montgomery D. R. Landslide erosion coupled to tectonics and river incision. Nat. Geosci. 5, 468–473 (2012).
-
- Whipple K. X. & Tucker G. E. Dynamics of the stream-power river incision model: Implications for height limits of mountain ranges, landscape response timescales, and research needs. J. Geophys. Res. Solid Earth 104, 17661–17674 (1999).
Publication types
LinkOut - more resources
Full Text Sources
Other Literature Sources