Foreign DNA capture during CRISPR-Cas adaptive immunity - PubMed
- ️Thu Jan 01 2015
. 2015 Nov 26;527(7579):535-8.
doi: 10.1038/nature15760. Epub 2015 Oct 21.
Affiliations
- PMID: 26503043
- PMCID: PMC4662619
- DOI: 10.1038/nature15760
Foreign DNA capture during CRISPR-Cas adaptive immunity
James K Nuñez et al. Nature. 2015.
Abstract
Bacteria and archaea generate adaptive immunity against phages and plasmids by integrating foreign DNA of specific 30-40-base-pair lengths into clustered regularly interspaced short palindromic repeat (CRISPR) loci as spacer segments. The universally conserved Cas1-Cas2 integrase complex catalyses spacer acquisition using a direct nucleophilic integration mechanism similar to retroviral integrases and transposases. How the Cas1-Cas2 complex selects foreign DNA substrates for integration remains unknown. Here we present X-ray crystal structures of the Escherichia coli Cas1-Cas2 complex bound to cognate 33-nucleotide protospacer DNA substrates. The protein complex creates a curved binding surface spanning the length of the DNA and splays the ends of the protospacer to allow each terminal nucleophilic 3'-OH to enter a channel leading into the Cas1 active sites. Phosphodiester backbone interactions between the protospacer and the proteins explain the sequence-nonspecific substrate selection observed in vivo. Our results uncover the structural basis for foreign DNA capture and the mechanism by which Cas1-Cas2 functions as a molecular ruler to dictate the sequence architecture of CRISPR loci.
Figures
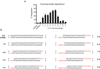
a, A plot of the percent integration of protospacers ± standard deviation with varying 3′ single-stranded DNA extensions. A representative gel is shown in Fig. 1a. b, Protospacer sequences used for the assays described in a and Fig. 1a, with the red nucleotides indicating the 3′ overhang regions.
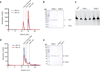
a, Gel filtration chromatogram of pre-assembled Cas1–Cas2 complex with protospacer DNA containing five nt 3′ overhangs. The dotted lines indicate the peak fractions of the Cas1–Cas2 complex without DNA, as shown in d. The solid lines indicate the peak fractions of the Cas1–Cas2 complex bound to DNA (first peak) and excess, unbound DNA (second peak). b, c, The fractions from Peak 1 (~12 ml) and Peak 2 (~15 ml) were analyzed by Coomassie-stained SDS-PAGE (b) and 12% urea-PAGE (c) to confirm the presence of Cas1, Cas2 and protospacer DNA. d, Gel filtration chromatogram of assembled Cas1–Cas2 without protospacer DNA. e, Coomassie-stained SDS-PAGE of the peak fractions from d. Supplementary Information contains the full images for b, c and e.
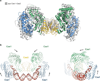
a, An overlay of the DNA bound Cas1–Cas2 structure with the apo Cas1–Cas2 (gray, PDB 4P6I). b, Vector lines depicting the conformational changes the Cas1–Cas2 complex undergoes upon protospacer DNA binding compared to the apo complex (PDB 4P6I). The Cas1 subunits rotate towards the direction of the arrows.
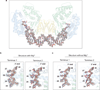
a, Simulated annealing Fo–Fc omit electron density map of the entire protospacer DNA using the “no Mg2+” map and model. b, c, Simulated annealing Fo–Fc omit electron density maps of the terminal five nucleotides in the active sites of the structures (a) with Mg2+ or (b) without Mg2+ in the crystallization condition. The maps are contoured at 2.0 σ.
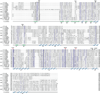
Sequence alignments of Cas1 from representative organisms with Type I CRISPR systems. The E. coli sequence is displayed at the top. The dots indicate the residues described in this study, with the red dots indicating the metal-binding residues. The box highlights the non-universal conservation of the E. coli Y22 residue in the β1 region of Type I CRISPR systems. The secondary structure representations shown are for the E. coli Cas1.
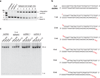
a, Representative agarose gel of in vitro integration reactions using increasing lengths of splayed ends. The average percent integration of three independent experiments is plotted in Fig. 3d. b, Sequences of protospacers used in the integration assays in a. c, A 12% denaturing polyacrylamide gel of protospacers after incubation with Cas1–Cas2 for 1 h at 37 °C in integration assay buffer conditions. The indicated DNA substrates are radiolabeled at the 5′ end. Supplementary Information contains the full images for a and c.
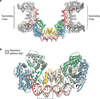
a, View of the symmetry mates (gray) contacting the non-catalytic Cas1 subunits (green). b, Superposition of our two crystal structures, with or without Mg2+, show a slight DNA kink in the structure bound to Mg2+ (dotted box). This region contacts α helix 7 of a symmetry mate, as described in the text.
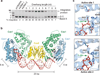
a, A representative agarose gel of in vitro integration reactions using increasing lengths of 3′ single-strand protospacer DNA overhangs. Percent integration values are the average of three independent experiments. b, The overall architecture of Cas1–Cas2 bound to protospacer DNA. The line segments indicate DNA regions lengths, spanning a total of 33 nt. c, Stick configurations of the two Cas1 active sites (blue subunits in b) that coordinate the nucleophilic 3′–OH ends of the protospacer (green arrow). Supplementary Information contains the full image for a.
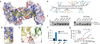
a, Electrostatic potential surface representation of the Cas1–Cas2 complex with the protospacer shown in yellow. b, Close up of the Arginine Channel that stabilizes the ssDNA overhang. c, Stick configuration representation of Arginine Clamp residues that coordinate the protospacer duplex region. d, Map of amino acid residues that coordinate the protospacer phosphodiester backbone (black dots). Residue colors indicate Cas1–Cas2 protomers from Fig. 1b. e, Agarose gels of in vivo spacer acquisition assays of Arginine Channel and Clamp mutant proteins. f, Plot of percent in vitro integration of either dsDNA (black) or 5 nt overhang (blue) protospacers with Cas1 WT, R59D or R66D complexed with Cas2. g, Fluorescence polarization binding assays of a 5 nt overhang protospacer with the same mutants in f complexed with Cas2. The calculated relative binding affinities (Kd) are indicated. Error bars represent the standard deviation of three independent experiments. Panel e–g data are results of minimally three biological replicates. Supplementary Information contains the full images for e.
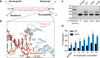
a, The 5 nt splayed protospacer sequence used for crystallization to determine the trajectory of the displaced non-nucleophilic strand. Cas1 Y22, involved in base stacking at the fork, is shown in blue. b, Close up of the DNA fork showing the base stacking interaction of Y22 with the terminal adenine nucleotide of the non-nucleophilic strand. The nucleotides are numbered from 5′ to 3′ of each DNA strand shown in a. The gray mesh shows the 2Fo–Fc density contoured at 2.2 σ of the first ejected nucleotide of the displaced strand. The arrows indicate the opposite trajectories of each strand. c, Agarose gel of in vivo acquisition assay of co-expressed Cas1 WT or the indicated Cas1 mutant with Cas2. Quantification is the mean of three independent experiments ± standard deviation. d, Plot of percent integration of increasing splayed nt at the protospacer ends using Cas1 WT (blue) or Y22A (blue) complexed with Cas2. Error bars represent the standard deviation of three independent experiments. Supplementary Information contains the full image for c.
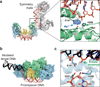
a, View of crystal packing from a symmetry mate complex (gray) showing coordination of the symmetry DNA along a Cas1 active site. The inset is a close up of the coordination of the phosphodiester backbone with metal-binding residues E141, H208 and D221. The mesh represents a Fo–Fc density for a Mg2+ ion, contoured at 2.2 σ. b, c, Model of protospacer DNA integration into target DNA (black) and positioning of the scissile phosphate (green arrow) and the 3′–OH nucleophile in the Cas1 active site.
Comment in
-
Structural biology: How CRISPR captures spacer invaders.
Attar N. Attar N. Nat Rev Microbiol. 2015 Dec;13(12):738-9. doi: 10.1038/nrmicro3585. Epub 2015 Nov 9. Nat Rev Microbiol. 2015. PMID: 26548917 No abstract available.
Similar articles
-
How type II CRISPR-Cas establish immunity through Cas1-Cas2-mediated spacer integration.
Xiao Y, Ng S, Nam KH, Ke A. Xiao Y, et al. Nature. 2017 Oct 5;550(7674):137-141. doi: 10.1038/nature24020. Epub 2017 Sep 4. Nature. 2017. PMID: 28869593 Free PMC article.
-
Integrase-mediated spacer acquisition during CRISPR-Cas adaptive immunity.
Nuñez JK, Lee AS, Engelman A, Doudna JA. Nuñez JK, et al. Nature. 2015 Mar 12;519(7542):193-8. doi: 10.1038/nature14237. Epub 2015 Feb 18. Nature. 2015. PMID: 25707795 Free PMC article.
-
Cas1-Cas2 complex formation mediates spacer acquisition during CRISPR-Cas adaptive immunity.
Nuñez JK, Kranzusch PJ, Noeske J, Wright AV, Davies CW, Doudna JA. Nuñez JK, et al. Nat Struct Mol Biol. 2014 Jun;21(6):528-34. doi: 10.1038/nsmb.2820. Epub 2014 May 4. Nat Struct Mol Biol. 2014. PMID: 24793649 Free PMC article.
-
CRISPR-Cas adaptation: insights into the mechanism of action.
Amitai G, Sorek R. Amitai G, et al. Nat Rev Microbiol. 2016 Feb;14(2):67-76. doi: 10.1038/nrmicro.2015.14. Epub 2016 Jan 11. Nat Rev Microbiol. 2016. PMID: 26751509 Review.
-
Adaptation in CRISPR-Cas Systems.
Sternberg SH, Richter H, Charpentier E, Qimron U. Sternberg SH, et al. Mol Cell. 2016 Mar 17;61(6):797-808. doi: 10.1016/j.molcel.2016.01.030. Epub 2016 Mar 3. Mol Cell. 2016. PMID: 26949040 Review.
Cited by
-
Kuznedelov K, Mekler V, Lemak S, Tokmina-Lukaszewska M, Datsenko KA, Jain I, Savitskaya E, Mallon J, Shmakov S, Bothner B, Bailey S, Yakunin AF, Severinov K, Semenova E. Kuznedelov K, et al. Nucleic Acids Res. 2016 Dec 15;44(22):10849-10861. doi: 10.1093/nar/gkw914. Epub 2016 Oct 13. Nucleic Acids Res. 2016. PMID: 27738137 Free PMC article.
-
Mechanisms of Type I-E and I-F CRISPR-Cas Systems in Enterobacteriaceae.
Xue C, Sashital DG. Xue C, et al. EcoSal Plus. 2019 Feb;8(2):10.1128/ecosalplus.ESP-0008-2018. doi: 10.1128/ecosalplus.ESP-0008-2018. EcoSal Plus. 2019. PMID: 30724156 Free PMC article. Review.
-
Wan H, Li J, Chang S, Lin S, Tian Y, Tian X, Wang M, Hu J. Wan H, et al. Sci Rep. 2019 Feb 28;9(1):3188. doi: 10.1038/s41598-019-39616-1. Sci Rep. 2019. PMID: 30816277 Free PMC article.
-
Cas1 and the Csy complex are opposing regulators of Cas2/3 nuclease activity.
Rollins MF, Chowdhury S, Carter J, Golden SM, Wilkinson RA, Bondy-Denomy J, Lander GC, Wiedenheft B. Rollins MF, et al. Proc Natl Acad Sci U S A. 2017 Jun 27;114(26):E5113-E5121. doi: 10.1073/pnas.1616395114. Epub 2017 Apr 24. Proc Natl Acad Sci U S A. 2017. PMID: 28438998 Free PMC article.
-
Disintegration promotes protospacer integration by the Cas1-Cas2 complex.
Ma CH, Javanmardi K, Finkelstein IJ, Jayaram M. Ma CH, et al. Elife. 2021 Aug 26;10:e65763. doi: 10.7554/eLife.65763. Elife. 2021. PMID: 34435949 Free PMC article.
References
-
- Barrangou R, et al. CRISPR provides acquired resistance against viruses in prokaryotes. Science. 2007;315:1709–1712. - PubMed
-
- Mojica FJ, Diez-Villasenor C, Garcia-Martinez J, Soria E. Intervening sequences of regularly spaced prokaryotic repeats derive from foreign genetic elements. Journal of molecular evolution. 2005;60:174–182. - PubMed
-
- Bolotin A, Quinquis B, Sorokin A, Ehrlich SD. Clustered regularly interspaced short palindrome repeats (CRISPRs) have spacers of extrachromosomal origin. Microbiology. 2005;151:2551–2561. - PubMed
-
- Pourcel C, Salvignol G, Vergnaud G. CRISPR elements in Yersinia pestis acquire new repeats by preferential uptake of bacteriophage DNA, provide additional tools for evolutionary studies. Microbiology. 2005;151:653–663. - PubMed
-
- Garneau JE, et al. The CRISPR/Cas bacterial immune system cleaves bacteriophage and plasmid DNA. Nature. 2010;468:67–71. - PubMed
Publication types
MeSH terms
Substances
LinkOut - more resources
Full Text Sources
Other Literature Sources
Molecular Biology Databases