Discovery and Functional Characterization of Diverse Class 2 CRISPR-Cas Systems - PubMed
- ️Thu Jan 01 2015
. 2015 Nov 5;60(3):385-97.
doi: 10.1016/j.molcel.2015.10.008. Epub 2015 Oct 22.
Omar O Abudayyeh 2 , Kira S Makarova 3 , Yuri I Wolf 3 , Jonathan S Gootenberg 2 , Ekaterina Semenova 4 , Leonid Minakhin 4 , Julia Joung 2 , Silvana Konermann 2 , Konstantin Severinov 5 , Feng Zhang 6 , Eugene V Koonin 7
Affiliations
- PMID: 26593719
- PMCID: PMC4660269
- DOI: 10.1016/j.molcel.2015.10.008
Discovery and Functional Characterization of Diverse Class 2 CRISPR-Cas Systems
Sergey Shmakov et al. Mol Cell. 2015.
Abstract
Microbial CRISPR-Cas systems are divided into Class 1, with multisubunit effector complexes, and Class 2, with single protein effectors. Currently, only two Class 2 effectors, Cas9 and Cpf1, are known. We describe here three distinct Class 2 CRISPR-Cas systems. The effectors of two of the identified systems, C2c1 and C2c3, contain RuvC-like endonuclease domains distantly related to Cpf1. The third system, C2c2, contains an effector with two predicted HEPN RNase domains. Whereas production of mature CRISPR RNA (crRNA) by C2c1 depends on tracrRNA, C2c2 crRNA maturation is tracrRNA independent. We found that C2c1 systems can mediate DNA interference in a 5'-PAM-dependent fashion analogous to Cpf1. However, unlike Cpf1, which is a single-RNA-guided nuclease, C2c1 depends on both crRNA and tracrRNA for DNA cleavage. Finally, comparative analysis indicates that Class 2 CRISPR-Cas systems evolved on multiple occasions through recombination of Class 1 adaptation modules with effector proteins acquired from distinct mobile elements.
Keywords: CRISPR-Cas adaptive immunity; Cas9; Cpf1; HEPN domain; PAM; RNA-seq; RuvC-like endonuclease; computational discovery pipeline; crRNA; tracrRNA.
Copyright © 2015 Elsevier Inc. All rights reserved.
Figures
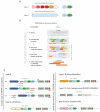
(A) Architectural principles of Class 1 (multi-protein effector complexes) and Class 2 (single-protein effector complexes) CRISPR-Cas systems. (B) Schematic of the computational pipeline for identification of putative new Class 2 loci. (C) Genomic architectures of the known and newly identified Class 2 CRISPR-Cas systems. The left panel shows the previously described three subtypes of type II and subtype V-A, and the right panel shows subtypes V-B and V-C, and type VI identified in this work. Subfamilies based on Cas1 are also indicated. The schematics include only the common genes represented in each subtype; additional genes present in some variants are omitted. The red rectangle shows the degenerate repeat. The gray arrows show the direction of CRISPR array transcription. PreFran, Prevotella-Francisella. See also Figures S1 and S2, and Table S1.
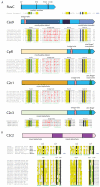
(A) Types II and V: TnpB-derived nucleases. The top panel shows the RuvC nuclease from Thermus thermophilus (PDB ID: 4EP5) with the catalytic amino acid residues denoted. Underneath each domain architecture, an alignment of the conserved motifs in selected representatives of the respective protein family (a single sequence for RuvC) is shown. The catalytic residues are shown by white letters on a black background; conserved hydrophobic residues are highlighted in yellow; conserved small residues are highlighted in green; in the bridge helix alignment, positively charged residues are in red. Secondary structure prediction is shown underneath the aligned sequences: H denotes α-helix and E denotes extended conformation (β-strand). The poorly conserved spacers between the alignment blocks are shown by numbers. See also Figures S3, S4 and S5. (B) Type VI: predicted RNases containing two HEPN domains. The top alignment blocks include selected HEPN domains described previously and the bottom blocks include the catalytic motifs from the putative type VI effector proteins. The designations are as in (A). See also Figures S3 and S6.
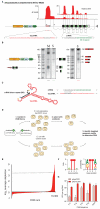
(A) RNA-sequencing shows the A. acideoterrestris C2c1 locus is highly expressed in the endogenous system, with processed crRNAs incorporating a 5’ 14-nt DR and 20-nt spacer. A putative 79-nt tracrRNA is expressed robustly in the same orientation as the cas gene cluster (see also Figures S7A, B and C). (B) Northern blot of RNAs expressed from the endogenous locus (M) and a minimal first-spacer array (S) show processed crRNAs with a 5’ DR and the presence of a small putative tracrRNA. Arrows indicate the probe positions and their directionality. (C) In silico co-folding of the crRNA direct repeat and putative tracrRNA shows stable secondary structure and complementarity between the two RNAs. 5’ bases are colored blue and 3’ bases are colored orange (see also Figure S7D). (D) Schematic of the PAM determination screen. (E) Depletion from the 5’ left PAM library reveals a 5’ TTN PAM. Depletion is measured as the negative log2 fold ratio and PAMs above a threshold of 3.5 are used to calculate the entropy score at each position. (F) Sequence logo for the AacC2c1 PAM as determined by the plasmid depletion assay. Left: Letter height at each position is measured by entropy scores and error bars show the 95% Bayesian confidence interval. Right: Letter height at each position is measured by the relative frequency of the nucleotide (see also Figure S7E). (G) Validation of the AacC2c1 PAM by measuring interference with 8 different PAMs. PAMs matching the TTN motif show depletion as measured by cfus.
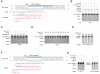
(A) Schematic of the AacC2c1 crRNA and tracrRNA design hybridizing to the EMX1 target site. (B) In vitro cleavage of the EMX1 target with the human cell lysate expressing AacC2c1 shows that in vitro targeting of AacC2c1 is robust and depends on tracrRNA. Non-targeting crRNA (crRNA 2) fails to cleave the EMX1 target, whereas crRNA 1 targeting EMX1 enabled strong cleavage in the presence of Mg++ and weak cleavage in the absence of Mg++. (C) In vitro cleavage of the EMX1 target in the presence of a range of tracrRNA lengths identifies the 78-nt species as the minimal tracrRNA form, with increased cleavage efficiency for the 91-nt form. (D) Analysis of the temperature dependency of the in vitro cleavage of the EMX1 target shows that the optimal temperature range of robust AacC2c1 cleavage is between 40°C and 55°C (E) In vitro validation of the AacC2c1 PAM requirements with four different PAMs. The PAMs matching the TTN motif are efficiently cleaved. (F) Schematic of the chimeric AacC2c1 sgRNA shown hybridized to the EMX1 DNA target with repeat:anti-direct pairing between segments derived from the tracrRNA (red) and the crRNA (green) (G) Comparison of the in vitro target cleavage in the presence of crRNA:tracrRNA AacC2c1 and sgRNA identifies comparable cleavage efficiencies.
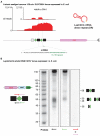
(A) RNA-sequencing of the Listeria seeligeria serovar 1/2b str. SLCC3954 C2c2 locus (see also Figures S7F and S7G). (B) Northern blot analysis of the Leptotrichia shahii DSM 19757 shows processed crRNAs with a 5’ DR. Arrows indicate the probe positions and their directionality.
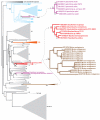
The tree was constructed from a multiple alignment of 1498 Cas1 sequences which contained 304 phylogenetically informative positions. Branches, corresponding to Class 2 systems are highlighted: cyan, type II; orange, subtype V-A; red, subtype V-B; brown, subtype V-C; purple, type VI. Insets show the expanded branches of the newly identified (sub)types. The bootstrap support values are given as percentage points and shown only for several relevant branches. The complete tree in the Newick format with species names and bootstrap support values and the underlying alignment are available at
ftp://ftp.ncbi.nih.gov/pub/wolf/_suppl/Class2/. See also Supplemental Experimental Procedures.
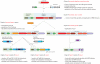
The scenario is a synthesis of the present and previous analyses (Makarova et al., 2011a; Makarova et al., 2013; Chylinski et al., 2014; Makarova et al., 2015). The Cas8 protein is hypothesized to have evolved by inactivation of Cas10 (shown by white X), which was accompanied by a major acceleration of evolution. Genes and portions of genes shown in gray denote sequences that are thought to have been encoded in the respective mobile elements but were eliminated in the course of evolution of CRISPR-Cas systems. Abbreviations: TR, terminal repeats; TS, terminal sequences; HD, HD family endonuclease; HNH, HNH family endonuclease; RuvC, RuvC family endonuclease; HEPN, putative endoribonuclease of HEPN superfamily.
Similar articles
-
The tracrRNA and Cas9 families of type II CRISPR-Cas immunity systems.
Chylinski K, Le Rhun A, Charpentier E. Chylinski K, et al. RNA Biol. 2013 May;10(5):726-37. doi: 10.4161/rna.24321. Epub 2013 Apr 5. RNA Biol. 2013. PMID: 23563642 Free PMC article.
-
The CRISPR-associated DNA-cleaving enzyme Cpf1 also processes precursor CRISPR RNA.
Fonfara I, Richter H, Bratovič M, Le Rhun A, Charpentier E. Fonfara I, et al. Nature. 2016 Apr 28;532(7600):517-21. doi: 10.1038/nature17945. Epub 2016 Apr 20. Nature. 2016. PMID: 27096362
-
C2c1-sgRNA Complex Structure Reveals RNA-Guided DNA Cleavage Mechanism.
Liu L, Chen P, Wang M, Li X, Wang J, Yin M, Wang Y. Liu L, et al. Mol Cell. 2017 Jan 19;65(2):310-322. doi: 10.1016/j.molcel.2016.11.040. Epub 2016 Dec 15. Mol Cell. 2017. PMID: 27989439
-
Biogenesis pathways of RNA guides in archaeal and bacterial CRISPR-Cas adaptive immunity.
Charpentier E, Richter H, van der Oost J, White MF. Charpentier E, et al. FEMS Microbiol Rev. 2015 May;39(3):428-41. doi: 10.1093/femsre/fuv023. Epub 2015 May 19. FEMS Microbiol Rev. 2015. PMID: 25994611 Free PMC article. Review.
-
Molecular Mechanisms of RNA Targeting by Cas13-containing Type VI CRISPR-Cas Systems.
O'Connell MR. O'Connell MR. J Mol Biol. 2019 Jan 4;431(1):66-87. doi: 10.1016/j.jmb.2018.06.029. Epub 2018 Jun 22. J Mol Biol. 2019. PMID: 29940185 Review.
Cited by
-
CRISPR-Cas, a robust gene-editing technology in the era of modern cancer immunotherapy.
Miri SM, Tafsiri E, Cho WCS, Ghaemi A. Miri SM, et al. Cancer Cell Int. 2020 Sep 15;20:456. doi: 10.1186/s12935-020-01546-8. eCollection 2020. Cancer Cell Int. 2020. PMID: 32973401 Free PMC article. Review.
-
The Functional Circular RNA Screening via RfxCas13d/BSJ-gRNA System.
Wu H, Chen LL. Wu H, et al. Methods Mol Biol. 2024;2765:173-191. doi: 10.1007/978-1-0716-3678-7_10. Methods Mol Biol. 2024. PMID: 38381340
-
The Development and Application of a Base Editor in Biomedicine.
Wang F, Zeng Y, Wang Y, Niu Y. Wang F, et al. Biomed Res Int. 2020 Aug 14;2020:2907623. doi: 10.1155/2020/2907623. eCollection 2020. Biomed Res Int. 2020. PMID: 32855962 Free PMC article. Review.
-
Pyne ME, Bruder MR, Moo-Young M, Chung DA, Chou CP. Pyne ME, et al. Sci Rep. 2016 May 9;6:25666. doi: 10.1038/srep25666. Sci Rep. 2016. PMID: 27157668 Free PMC article.
-
CRISPR-Based Diagnosis of Infectious and Noninfectious Diseases.
Jolany Vangah S, Katalani C, Booneh HA, Hajizade A, Sijercic A, Ahmadian G. Jolany Vangah S, et al. Biol Proced Online. 2020 Sep 14;22:22. doi: 10.1186/s12575-020-00135-3. eCollection 2020. Biol Proced Online. 2020. PMID: 32939188 Free PMC article. Review.
References
-
- Barrangou R, Fremaux C, Deveau H, Richards M, Boyaval P, Moineau S, Romero DA, Horvath P. CRISPR provides acquired resistance against viruses in prokaryotes. Science. 2007;315:1709–1712. - PubMed
Publication types
MeSH terms
Substances
Grants and funding
LinkOut - more resources
Full Text Sources
Other Literature Sources
Research Materials
Miscellaneous