Effects of electrical stimulation on rat limb regeneration, a new look at an old model - PubMed
- ️Thu Jan 01 2015
Effects of electrical stimulation on rat limb regeneration, a new look at an old model
Liudmila P Leppik et al. Sci Rep. 2015.
Abstract
Limb loss is a devastating disability and while current treatments provide aesthetic and functional restoration, they are associated with complications and risks. The optimal solution would be to harness the body's regenerative capabilities to regrow new limbs. Several methods have been tried to regrow limbs in mammals, but none have succeeded. One such attempt, in the early 1970s, used electrical stimulation and demonstrated partial limb regeneration. Several researchers reproduced these findings, applying low voltage DC electrical stimulation to the stumps of amputated rat forelimbs reporting "blastema, and new bone, bone marrow, cartilage, nerve, skin, muscle and epiphyseal plate formation". In spite of these encouraging results this research was discontinued. Recently there has been renewed interest in studying electrical stimulation, primarily at a cellular and subcellular level, and studies have demonstrated changes in stem cell behavior with increased proliferation, differentiation, matrix formation and migration, all important in tissue regeneration. We applied electrical stimulation, in vivo, to the stumps of amputated rat limbs and observed significant new bone, cartilage and vessel formation and prevention of neuroma formation. These findings demonstrate that electricity stimulates tissue regeneration and form the basis for further research leading to possible new treatments for regenerating limbs.
Figures
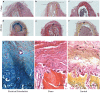
All sections were stained with AB&OG. Approximate level of the amputation site is indicated with black dotted lines (a–f). Top row are electrically stimulated (a), sham (b) and control (c) stumps at 7 days after amputation (scale bar = 500 μM). Middle row are electrically stimulated (d), sham (e) and control (f) stumps at 28 days after amputation. Bottom row are high magnification (20x) (scale bar = 50 μM) of electrically stimulated (g), sham (h) and control (j) selected sites (black box) on stumps at 28 days after amputation. Significant cartilage formation is seen in the electrically stimulated (g) histological section versus scar formation in the sham (h) and control (j) sections.
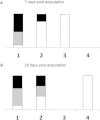
Osteocartilaginous formation in electrically stimulated (white), sham (gray) and control (black) stumps at 7 (a) and 28 (b) days after amputation. Scores derived from a scale of +1 to +4, where +1 = no growth; +2 = growth <100 μM; +3 = growth between 100 and 1,000 μM, and +4 = growth >1,000 μM.
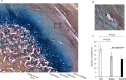
(a) Osteochondral ossification and vascularization (AB&OG staining, 20x, scale bar = 100 μM). Vascular ingrowth (black arrows). Reserve, proliferating cartilage, hypertrophy, calcification and newly formed bone areas are visible. (b) High magnification (40×; scale bar = 10 μM) of new vessels. (c) Graph indicating number of new vessels in 3 × 1 mm2 square areas in rat limb stumps in all 3 groups 28 days post amputation. Significantly more new vessels are visible in electrically stimulated compared to sham (p < 0,05) and control (p < 0.01) stumps.
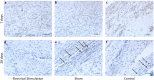
(Neurofilament protein staining, 20× magnification, scale bar = 50 μM). (a–c) No signs of neuroma formation in electrically stimulated (a), sham (b) and control (c) stumps 7 days post amputation. Neuroma formation (arrows) in sham (e) and control (f) stumps and no neuroma formation in electrically stimulated stump tissue (d) 28 days post amputation.
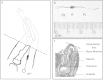
(a) Drawing of rat limb amputation with the amputation site (black dotted line) and implanted electrical stimulation device (DES). (b) Photo of the electrical stimulation device with a silver wire electrode loop (Ag) and platinum wire electrode (Pt) interposed with a 10 MΩ resistor (R). (c) Photo of longitudinal histological section of amputated limb stump, with the amputation site (black dotted line) and labeled bone marrow, muscle, humerus and scapula bones.
Similar articles
-
Response of amputated rat limbs to fetal nerve tissue implants and direct current.
Sisken BF, Fowler I, Romm S. Sisken BF, et al. J Orthop Res. 1984;2(2):177-89. doi: 10.1002/jor.1100020209. J Orthop Res. 1984. PMID: 6491811
-
Regeneration potency of mouse limbs.
Masaki H, Ide H. Masaki H, et al. Dev Growth Differ. 2007 Feb;49(2):89-98. doi: 10.1111/j.1440-169X.2007.00909.x. Dev Growth Differ. 2007. PMID: 17335430
-
Cannata SM, Bagni C, Bernardini S, Christen B, Filoni S. Cannata SM, et al. Dev Biol. 2001 Mar 15;231(2):436-46. doi: 10.1006/dbio.2001.0161. Dev Biol. 2001. PMID: 11237471
-
Some principles of regeneration in mammalian systems.
Carlson BM. Carlson BM. Anat Rec B New Anat. 2005 Nov;287(1):4-13. doi: 10.1002/ar.b.20079. Anat Rec B New Anat. 2005. PMID: 16308859 Review.
-
Alibardi L. Alibardi L. Prog Histochem Cytochem. 2014 Jan;48(4):143-244. doi: 10.1016/j.proghi.2013.12.001. Epub 2014 Jan 1. Prog Histochem Cytochem. 2014. PMID: 24387878 Review.
Cited by
-
Churchill CDM, Winter P, Tuszynski JA, Levin M. Churchill CDM, et al. iScience. 2019 Jan 25;11:42-56. doi: 10.1016/j.isci.2018.12.003. Epub 2018 Dec 11. iScience. 2019. PMID: 30590250 Free PMC article.
-
Variable Range Hopping in SrTiO3-Ca10(PO4)6(OH)2 Bio-Ceramic Composites.
Das A, Dobbidi P. Das A, et al. ACS Omega. 2021 Oct 3;6(40):25916-25925. doi: 10.1021/acsomega.1c02273. eCollection 2021 Oct 12. ACS Omega. 2021. PMID: 34660954 Free PMC article.
-
Electrical Stimulation Decreases Dental Pulp Stem Cell Osteo-/Odontogenic Differentiation.
Oliveira KMC, Leppik L, Keswani K, Rajeev S, Bhavsar MB, Henrich D, Barker JH. Oliveira KMC, et al. Biores Open Access. 2020 May 27;9(1):162-173. doi: 10.1089/biores.2020.0002. eCollection 2020. Biores Open Access. 2020. PMID: 32642331 Free PMC article.
-
Biophysical control of plasticity and patterning in regeneration and cancer.
Murugan NJ, Cariba S, Abeygunawardena S, Rouleau N, Payne SL. Murugan NJ, et al. Cell Mol Life Sci. 2023 Dec 15;81(1):9. doi: 10.1007/s00018-023-05054-6. Cell Mol Life Sci. 2023. PMID: 38099951 Free PMC article. Review.
-
Tyler SEB. Tyler SEB. Front Physiol. 2017 Sep 4;8:627. doi: 10.3389/fphys.2017.00627. eCollection 2017. Front Physiol. 2017. PMID: 28928669 Free PMC article. Review.
References
-
- Ziegler-Graham K. MacKenzie E. J., Ephraim P. L., Travison T. G. & Brookmeyer R. Estimating the prevalence of limb loss in the United States: 2005 to 2050, Arch Phys Med Rehabil 89, 422–429 (2008). - PubMed
-
- Petruzzo P. & Dubernard J. M. World experience after more than a decade of clinical hand transplantation: update on the French program, Hand Clin 27, 411–6, vii (2011). - PubMed
-
- Shores J. T., Brandacher G., Schneeberger S., Gorantla V. S. & Lee W. P. Andrew. Composite tissue allotransplantation: hand transplantation and beyond, J Am Acad Orthop Surg 18, 127–131 (2010). - PubMed
Publication types
MeSH terms
LinkOut - more resources
Full Text Sources
Other Literature Sources